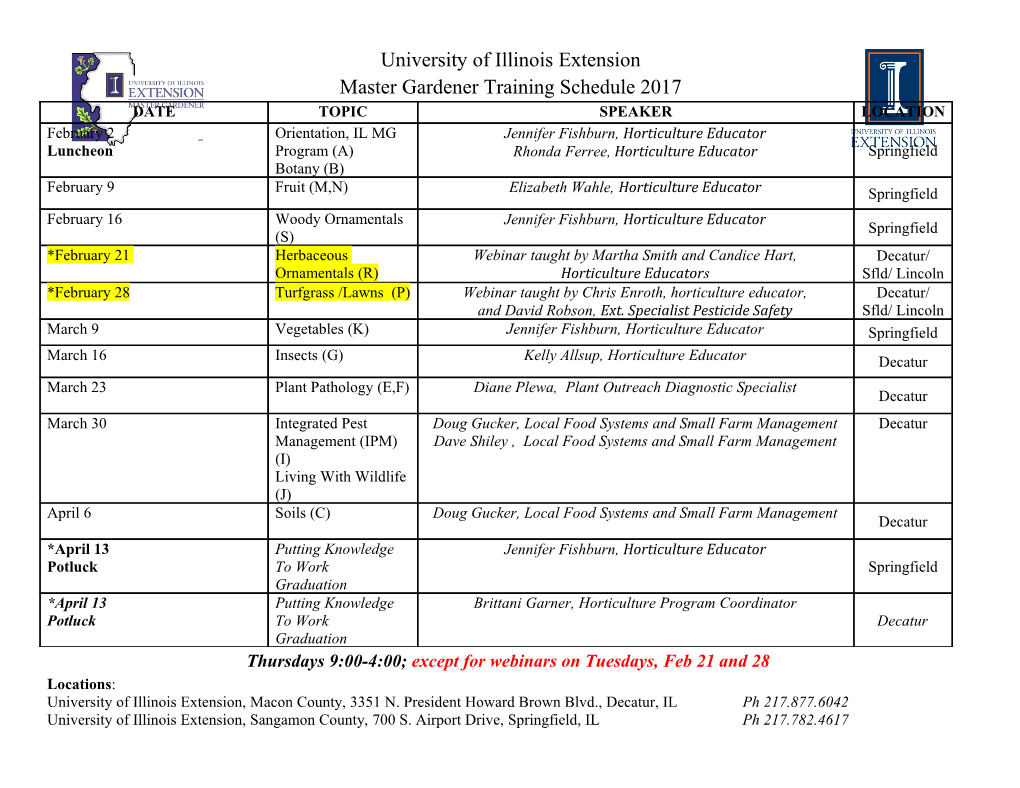
Variation in sound production by the Pot-bellied Seahorse, Hippocampus abdominalis, during feeding A thesis submitted to the Graduate School of the University of Cincinnati in partial fulfillment of the requirements for the degree of Master of Science in the Department of Biological Sciences of the College of Arts and Sciences by Brittany A. Hutton B.S. Miami University August 2017 Committee Chair: Pete Scheifele, Ph.D LCDR USN (Ret) Cincinnati, OH ABSTRACT The Pot-bellied seahorse, Hippocampus abdominalis, produces acoustic signals during feeding behavior. The acoustic signal, referred to as a “click”, is typically accompanied by a head-motion called a “snick”, and both occur during foraging by seahorses. I determined that clicks are usually associated with snicks (although not always) , supporting the “rub-knock” stridulation mechanism of sound production common in seahorses. I examined variation in peak frequency (Hz) as well as peak intensity (Watts/m2) of feeding clicks, but found no significant difference between sexes or between adults and juveniles. Analyses of clicking in light and dark environments revealed that seahorses click much more in the light and exhibit an increased number of clicks in the presence of food. Female seahorses clicked significantly more often than males, suggesting a possible role for sexual selection on acoustic signals in this sex-role reversed species. A body condition index (BCI) of residual square root area (cm2) was inversely correlated with mean peak frequency (Hz) of feeding clicks, suggesting the acoustic signal may contain information on mate size and quality. These results suggest that the function of sound production by seahorses during feeding is related to conspecific communication. ii iii Acknowledgements: Over the course of my education as a graduate student here at the University of Cincinnati I have had the fortunate experience of working with and being supported by many advisors, educators and colleagues. I’d like to extend my gratitude to the Newport Aquarium for allowing me to conduct the entirety of my study on site with the use of their seahorses. First and for most I would like to thank both of my advisors, Dr. Pete Scheifele and Dr. George Uetz for their support of my education and research in the Department of Biological Sciences. Dr. Pete Scheifele not only encouraged my interest in animal bioacoustics and work in animal welfare but opened lines of communication to allow me to conduct the entirety of my research projects through the Newport Aquarium. Dr. Gerorge Uetz was crucial in the experimental design of my projects and was consistently a wonderful source for insight into animal behavior and scientific writing. I would like to also extend my heartfelt thanks to my other committee member Dr. John Layne who took time to coach me through the sensory physiology of my model system. The graduate students of the Uetz Lab were all extremely supportive during my time here. I would especially like to thank Brent Stoffer, Alex Sweger, Rachel Gilbert, Tim Meyer, Emily Pickett, Maddi Lallo and Trinity Waals for all their continued support and advice. To the many other graduate students in the Department of Biological Sciences I thank you for making my time here at the University of Cincinnati so enjoyable. I’d like to thank the FETCHLABTM and all of its members for not only support of my research but also providing the necessary equipment for me to conduct my studies. Finally, I would like to extend a personal thank you to my fiancé Christian Romanchek for all his love and support. To my parents, sisters and brothers I’d like to say thank you for providing the necessary escape from school life and the always gentle reminder to not worry so much and just enjoy myself and my time in graduate school. iv Table of Contents Abstract ii Acknowledgements iv List of Tables & Figures vii General Introduction 1 Study Organism 6 Objectives and Hypotheses 6 Chapter I. Characterization of sound production by the Pot-bellied seahorse (Hippocampus abdominalis) during feeding 13 Abstract 14 Introduction 15 Methods 16 Results 18 Discussion 20 References 23 Tables and Figures 25 II. Contexts of Sound Production 33 Abstract 34 Introduction 34 v Methods 36 Results 38 Discussion 38 References 40 Tables and Figures 44 III. Other Considerations- Function of Sound Production in Seahorses 47 Introduction 47 References 53 vi List of Figures & Tables: Figure 1.1: Scatterplot of feeding clicks and snicks Figure 1.2: Spectrogram Figure 1.3: Power spectrum of the baseline noise level Figure 1.4: Power spectrum for feeding click Figure 1.5: Mean Peak Frequency (Hz) of feeding clicks Figure 1.6: Mean Peak Intensity (Watts / m2) of feeding clicks Figure 1.7: Mean RMS Level (Watts / m2) of feeding clicks Figure 1.8: Mean number of feeding clicks between sexes, adults, juveniles Figure 2.1: Bar graph of number of feeding clicks in Light and Dark within Food and No Food Figure 2.2: Scatterplot of Seahorse Standard Length (Hz) and Mean Peak Frequency (Hz) Figure 2.3: Peak Frequency (Hz) x Residual BCI (Body Condition Index) vii GENERAL INTRODUCTION Communication can be defined as an exchange of information between a sender and a receiver, wherein the receiver will process the information from the signal produced by the sender and then respond accordingly (Bradbury & Vehrencamp, 2011). Animal communication uses a multitude of signaling modalities which can be varied depending on both the context in which the signal is relayed for an intended receiver or the environment (Stevens, 2013). These signals can be very complex and include multiple modalities (Partan & Marler, 1999). Communication has a variety of modes from which it can be transferred. Communication can take the form of vibrational or acoustic cues (vocalizations), visual cues (color displays) or chemical cues (pheromones) (Bradbury & Vehrencamp, 2011; Stevens, 2013; Partan & Marler, 1999). Visual cues may include a coloration or “body language” that sends a signal to another individual such as in courtship between guppies (Endler, 1987) and swordtail fish (Morris & Hankison, 2001; Basolo, 1990). Chemical cues are also used in courtship such as the pheromones in spider silk (Pollard et.al, 2012). Acoustic cues are used in many contexts such as courtship, predator-prey interactions and territorial defense (Bradbury & Vehrencamp, 2011). When one or more of these modes are used to convey information, it is called multi-modal communication (Partan & Marler, 1999) such as the use of visual and vibratory cues used in courtship by the wolf spider, Schizocosa ocreata (Taylor et.al 2005, Hebets & Uetz, 1999). Acoustic communication is among the best studied modes (Bradbury & Vehrencamp, 2011). Many animals use vocalizations or sound in communication. Many species of birds use songs in courtship or territorial defense (Thorpe, 1961). Crickets will also produce sound via stridulatory organs (file-scraper mechanism) (Gerhardt & Huber, 2002). Sound is defined as the vibration of molecules as a wave propagates through a medium. Sound waves are characterized 1 by direction, speed, amplitude (loudness) and frequency (pitch) (Gerhardt, 1992; Gerhardt, 1998). Sound waves can be digitized to visualize as a spectrogram or waveform for easier analysis. During communication, these properties of acoustic signals are perceived and analyzed by the receiver of the sound (Gerhardt, 1992). Acoustic signals are received via mechanoreceptors. These receptors can sense vibration either airborne (sound) or through the substrate (seismic). Seismic-borne vibration can be received via specialized structures on the animals; for spiders, these signals are received by the slit sensilla and lyriform organs (Barth, 2004). Airborne sound signals are received by the animal ear. The sound is transmitted into the ear and to hair-cells that will bend in response to the signal depending on the frequency of the sound (Davis, 1957). These hair-cells start a cascade of action potentials transmitting information so that the animal can assess the signal and respond accordingly (Davis, 1957). Acoustic signals that are transported through water are perceived by different mechanisms depending on the aquatic animal receiving the sound. Sound waves behave very differently across terrestrial and aquatic environments. The speed of sound in air is 343 m/s while the speed of sound in water is 1482 m/s; due to the higher density of water as a medium. The increased speed of sound in water results in sound waves with a much higher wavelength, which can therefore travel much further a property used by many baleen whales during conspecific communication (Edds-Walton, 2012). Due to these differences in sound wave propagation, terrestrial and aquatic animals differ in the way they communicate acoustically. Terrestrial animals typically communicate acoustically by using vocal folds that vibrate with airflow (Ladich & Winkler, 2017). While some aquatic animals produce sound with modified vocal folds outfit for communication over long distances (cetaceans); fish produce and 2 receive sound via different mechanisms that are best for short-distance communication (Ladich & Winkler, 2017). The unique properties of sound waves in water influence acoustic communication by marine and freshwater animals. Aquatic species use various sound perception and production mechanisms. Odontocetes (Toothed whales) receive sound waves from the lower jaw, this signal is then conducted through mandibular fat bodies to the middle ear then auditory brain for processing (Ladich & Winkler, 2017). Fish are thought to receive sound via the otolith (Ladich & Winkler, 2017), the lateral line (Coombs et.al, 2014) and in some cases the swim bladder (Ladich & Winkler, 2017). There is controversy surrounding whether or not sound reception by the otolith and lateral line are always equivalent in their detection efforts or if they can also work independently in signal reception (Coombs et.al 2014). Both the inner ear and lateral line systems use hair cells in signal transduction.
Details
-
File Typepdf
-
Upload Time-
-
Content LanguagesEnglish
-
Upload UserAnonymous/Not logged-in
-
File Pages63 Page
-
File Size-