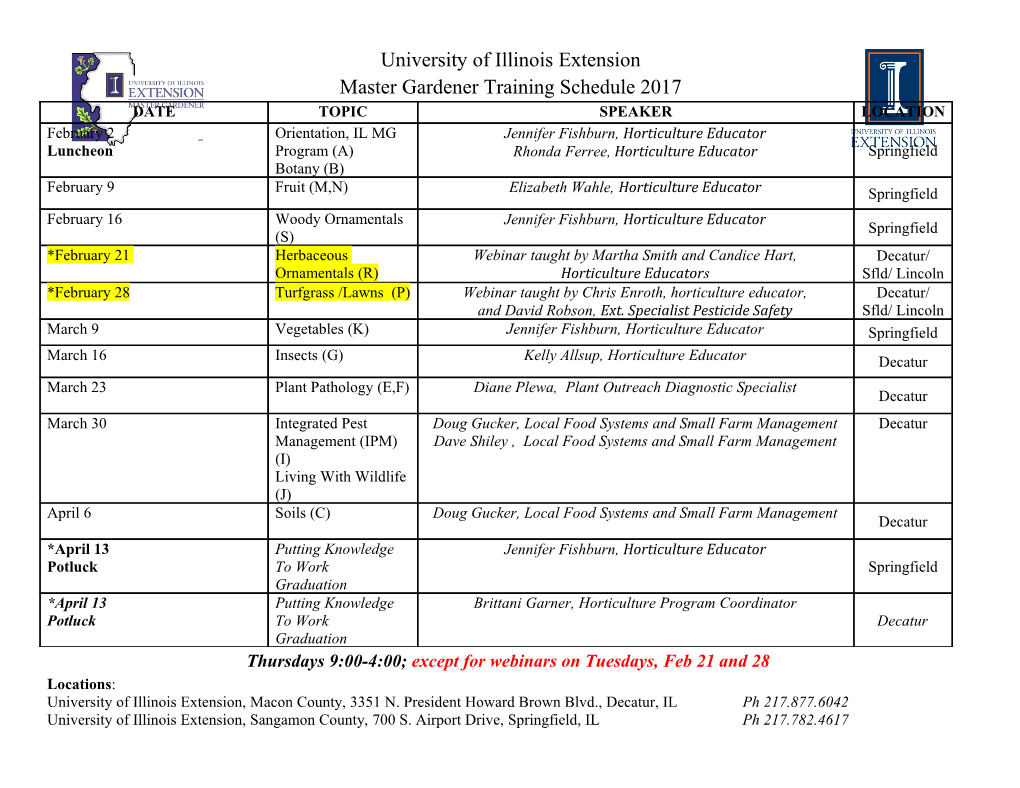
Model studies on the effects of composition differences of direct reduction pellets and an adaptive addition of slag formers for the EAF process Björn Keskitalo Master of Science Thesis Version 0.9429 2015-04-29 Supervisors Magnus Tottie, LKAB Niloofar Arzpeyma, Kobolde & Partners Examiner Pär Jönsson, KTH Abstract This work has been conducted to study the effect of different types of iron ore pellets on an EAF based steel production. The study has focused on how the chemical composition of the raw materials influences the slag amount and as a result the EAF operation. It is also shown that the raw material selection can be optimized for a better slag practice. The advantage of slag former additions that are strictly adapted to the EAF charge composition is also demonstrated. This work is based on a MgO-saturation model for slag, developed by Dr Roger Selin. The model has been implemented in RAWMATMIX®, a software developed by Kobolde & Partners AB in Stockholm. In this report I discuss the following studies: i) A study and comparison between different basicity indices and MgO-saturation for EAF slags, ii) a comparison between different DR-pellets and mixes between them and their corresponding DRI, iii) a parameter study on how different properties for the DR-pellet and DRI influence the EAF process, and iv) a case study of two hypothetical steel plants to illustrate the value of an adaptive slag former addition based on the raw material input. Overall, these studies describe the value of using DRI based on DR-pellets, containing low amount of acidic oxides and balanced amounts of MgO, for an EAF based steel production. Table of Content 1. Introduction 1.1 Introduction Page 1 1.2 Purpose Page 1 2 Background 2.1 Direct Reduced Iron Page 2 2.2 EAF Process Page 4 2.3 EAF Slag Page 5 2.4 MgO-saturation model Page 6 2.5 Calculation tool Page 7 3. Execution, Limitations and Input 3.1 Setup and work order Page 8 3.2 Limitations Page 11 3.3 Input Page 11 4. Results and Discussion 4.1 Basicity and MgO-saturation Page 17 4.2 Basicity and slag forming fractions Page 18 4.3 DR/DRI Comparison Page 20 4.4 Parameter study Page 23 4.5 Value-in-use Page 26 5. Conclusions Page 30 6 Acknowledgment Page 32 7. References Page 33 Appendix 1 – DR/DRI Page 34 Appendix 2 – EAF slag Page 45 Appendix 3 – Input Page 52 1.1 Introduction The market for iron and steel produced using direct reduction (DR) of iron ore has been estimated to grow and is becoming of more importance for the iron and steel industry. Today, LKAB develops and produces iron ore pellets for DR. The pellets produced contain low amounts of silicon and aluminum, which results in smaller slag amounts when melted in an electric arc furnace (EAF). In order to improve the properties during DR and melting, dolomite is added to the pellets. Dr Roger Selin has developed a model to calculate the MgO solubility and equilibrium distributions for phosphorous and vanadium for slag compositions from direct reduced iron (DRI). This model has been implemented in a web-based optimization program, RAWMATMIX® by Kobolde & Partners AB. 1.2 Purpose 1. Model Focus: Study the chemistry of pellets for DR by using RAWMATMIX® and identify the advantages and disadvantages of using different DR pellets as raw materials in electric arc furnaces. 2. Customer Focus: How the model and results could be presented to LKAB's customers and be used by them, in order to achieve an efficient pellet selection and EAF operation. 1 2. Background 2.1 Direct Reduced Iron Direct reduced iron (DRI) is a raw material used in steel production. DRI is primarily used in EAF when there is a demand for low amounts of metallic impurities, such as copper and tin. It is also used when there is a shortage of suitable scrap. Direct reduction (DR) is a solid state type of reduction of iron ore to metallic iron by the use of a reducing atmosphere or environment [1,2,3]. The most common way to produce DRI for EAF based steel production is by DR of a certain grade of pellets, direct reduction pellets (DR-pellets). These are typically produced in vertical direct reduction shaft furnaces (DR-furnaces). The DR-pellet, compared to pellets designed for a blast-furnace use, must have lower amounts of silica, sulfur and other impurities to be used in an EAF [1,2,3]. In a DR-furnace, the reduction takes place as the DR-pellets flow downwards from the top to the bottom of the furnace. This is done as the reduction gas at a high temperature flow upwards from the inlet of the reduction zone to the top of the DR-furnace. The reduction gas is injected at the middle of the furnace just above the cooling zone for the DRI at the bottom end of the DR-furnace. In the cooling zone, a carbon rich gas is injected to increase the carbon content of the DRI inside the furnace and also to cool down the DRI to a desired temperature. This means that it is possible to control both the carbon content of the DRI and the metallization, which is the ratio of metallic iron to the total mass of iron. This is done by changing the process conditions, such as the production rate for the DR- furnace. An overview of the process can be seen in Figure 1, which illustrates a Midrex process [1,2,3,4]. Another common process is the Energiron process (HYL) [5]. The reduction gas consists primarily of carbon-monoxide (CO) and hydrogen-gas (H2). The reduction gas is normally produced by a reformation of natural gas. The way that the natural gas is reformed is one major difference between different types of DR processes [2,3,6,7]. The following reactions take place within a DR-furnace [2,3,4,6,7]: 1: 3Fe2O3 + H2 = 2Fe3O4 + H2O 2: 3Fe2O3 + CO = 2Fe3O4 + CO2 3: Fe3O4 + H2 = 3FeO + H20 4: Fe3O4 + CO = 3FeO + CO2 5: FeO + H2 = Fe + H2O 6: FeO + CO = Fe + CO2 2 Figure 1. An illustration of a DR-furnace of a Midrex type. It shows an overview of the zones and the reactions within a DR-furnace [1]. DRI is produced in the following three major categories: 1) Cold DRI is the most common DRI product and is charged into an EAF at ambient temperature, 2) hot DRI is designed to be used in a nearby EAF to utilize the latent-heat in hot fresh DRI, and 3) hot briquetted iron, which is a compressed DRI type with the purpose to reduce the surface area, and thereby the reactivity, of the DRI. Also, it is produced to enable longer transportation and storage time [1,2,3]. A problem that might occur during DR of DR-pellets in a DR-furnace is that the DR-pellets start to stick to each other, which will form clusters. This happens in the reduction zone in the DR-furnace where the temperature and metallization of the DRI is high. To avoid this type of behavior it is common to coat the DR-pellets with an oxide which has a high melting temperature, such as CaO or MgO[2,3]. Another important aspect of the DR of DR-pellets is that all the slag elements remain and follows the pellet on its transition from iron ore to metallic iron. This is due to the fact that the DR is a solid state reaction during which primarily the iron oxide is reduced while almost all the other elements and 3 compounds are kept intact. Therefore, the slag components can either be removed before the pellet is produced or follow the pellet until it is melted, which for most DRI types are in an EAF [1,2,3]. 2.2 EAF Process The electric arc furnace (EAF) is primarily a melting furnace, which produces liquid steel by melting scrap and DRI. Today, the typical tap-to-tap time is around 40-60 min. In 2011, EAFs produced over 25% of the world's total crude steel production. A typical layout of an EAF can be seen in Figure 2. The charge is melted by powerful electric arcs, which are formed between the electrodes and the charge. As these arcs radiate a lot of heat against the furnace walls and roof, the walls and roof often contain cooling elements to increase the durability. Foaming slag is also an important tool which is used to protect the walls and roof [8,9,10]. Figure 2. Standard design of a three-phase AC EAF. And the number shows the following parts of the EAF: 1) transformer, 2) cable connection, 3) electrodes, 4) electrode clamps, 5) arms, 6) off-gas duct, 7) cooled wall panels, 8) structure, 9) basculating structure, 10) rack, 11) cooled roof, 12) basculating device, 13) hydraulic group [8]. Although most of the energy in an EAF comes from electric power, it is also important to use chemical energy to minimize the tap-to-tap times and the consumption of electrical energy. The chemical energy is often based on oxidation of carbon. The carbon comes from either the raw material, i.e. DRI or is injected into the furnace during the melting. Injection of carbon and oxygen into the slag also contribute to a foaming of the slag [8,9,10]. 2.3 EAF Slag 4 The EAF slag is an ionic solution that floats on top of the steel.
Details
-
File Typepdf
-
Upload Time-
-
Content LanguagesEnglish
-
Upload UserAnonymous/Not logged-in
-
File Pages68 Page
-
File Size-