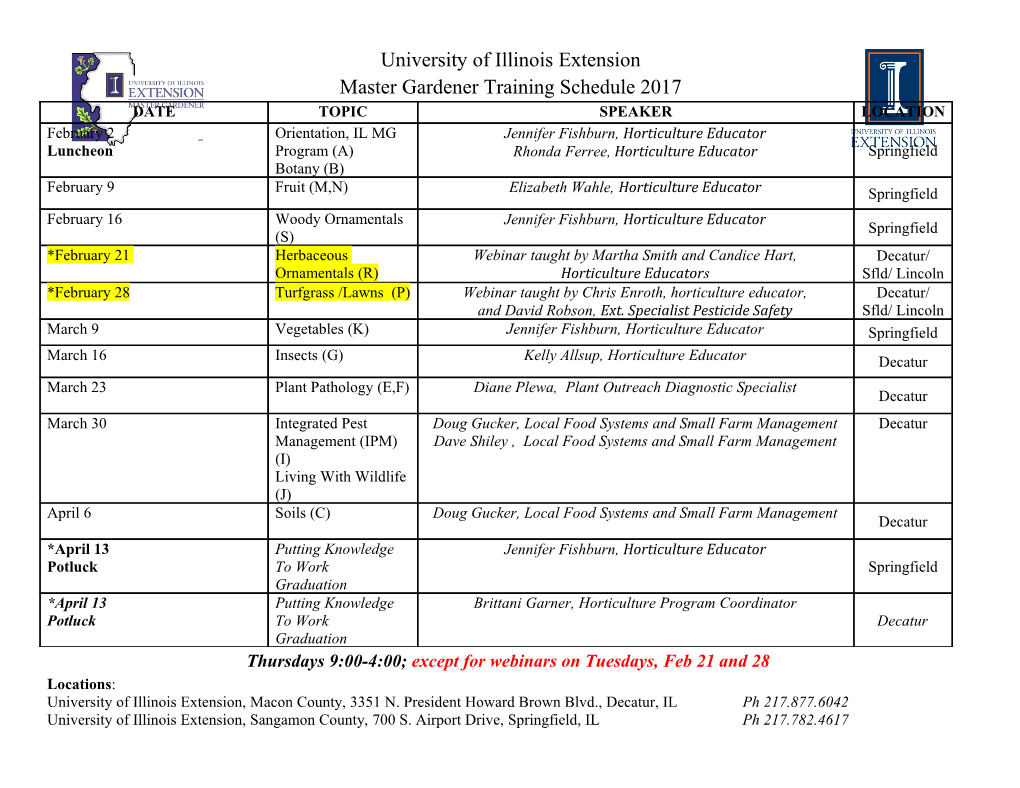
Chapter 5 The Working Cell: Chemical Energetics and Enzymes I. Energy: The capacity to do work. The ability to change matter Can exist in two forms: 1. Kinetic energy: Energy of motion. Energy that is actively performing work. Examples: Heat: Energy of particles in motion. Light: Energy of photons of light 2. Potential energy: Stored energy due to position or arrangement of matter. Examples: Chemical energy: Potential energy of molecules due to the arrangement of atoms. The most important type of energy for living organisms. Position: Bicycle at the top of a hill. Kinds of Energy Chemical Nuclear Electrical Electromagnetic Light Mechanical Heat Sound II. Energy Transformation Energy can be converted from one kind to another. Transformations are inefficient, generating heat. Examples: Light energy -------- --> Chemical energy (sugar) + Heat Chemical energy -- ---> Mechanical energy + Heat Electrical energy --- --> Light energy + Heat Chemical energy --- --> Biological work + Heat Heat is easily measured energy, because all other forms of energy can be converted to heat. From a biological standpoint, heat is a poor kind of energy which is not very useful to do work. Why? Because heat is lost to the environment. III. All energy transformations are subject to the First and Second Laws of Thermodynamics 1. First Law of Thermodynamics: Energy can be transformed (e.g.: chemical to mechanical), but cannot be created nor destroyed. The total amount of energy in the universe is constant. Biological Consequence: Living organisms cannot create the energy they need to live. They must capture it from their environment. Sources of energy used by living organisms: Sun and chemical energy. 2. Second Law of Thermodynamics ENERGY CONVERSIONS ARE INEFFICIENT In any energy transformation, a certain amount of energy is lost as heat. By comparison, living organisms are relatively efficient. Electrical Energy -------> ---------> 5% Light + 95% Heat Chemical Energy --> ----------------> 25% Mechanical (Gasoline) 75% Heat Chemical Energy --------> -----------> 40% ATP + 60% Heat (Glucose) III. Laws of Thermodynamics (Cont.) 2. Second Law of Thermodynamics: The universe inevitably tends toward a state of increased disorder or chaos (entropy). For this reason, energy transformations are inefficient. Entropy (S): Measure of disorder. Disorganized, less usable energy (heat). Only way to overcome entropy, is to put energy into the system. Biological Consequences: Living organisms must constantly take in energy to avoid entropy (disintegration, death and decay). High quality energy is a limited resource, because usable energy, decreases over time. IV. Chemical Reactions Either Store or Release Energy: I. Exergonic Reactions: Release free energy. Also exothermic (release heat). Products have less energy than the reactants. Example: Cellular respiration is an exergonic process: C6H12O6 + 6 O2 ----> 6 CO2 + 6 H2O + Energy Sugar Oxygen Carbon Water Dioxide High Energy Reactants Low Energy Products IV. Chemical Reactions Store or Release Energy: II. Endergonic Reactions: Require net input of free energy. Also endothermic (absorb heat). Products have more energy than the reactants. Create products that are rich in potential energy. Example: Photosynthesis is an endergonic process: 6 CO2 + 6 H2O + Sunlight ----> C6H12O6 + 6 O2 Carbon Water Energy Sugar Oxygen Dioxide Low Energy Reactants High Energy Products Chemical Reactions Either Store or Release Energy Endergonic Reactions Exergonic Reactions Require Energy Release Energy Higher Energy Products Lower Energy Products Metabolism: All chemical processes that occur within a living organism. Either catabolic or anabolic reactions. I. Catabolic Reactions: Release energy (exergonic). Break down large molecules (proteins, polysaccharides) into their building blocks (amino acids, simple sugars). Often coupled to the endergonic synthesis of ATP. Examples: 1. Cellular respiration is a catabolic process: C6H12O6 + 6 O2 -------> 6 CO2 + 6 H2O + Energy Sugar Oxygen Carbon dioxide Water 2. The digestion of sucrose is a catabolic process: Sucrose + Water -------> Glucose + Fructose + Energy Disaccharide Monosaccharides Metabolism: Catabolism + Anabolism II. Anabolic Reactions: Require energy (endergonic). Build large molecules (proteins, polysaccharides) from their building blocks (amino acids, simple sugars). Often coupled to the exergonic breakdown or hydrolysis of ATP. Examples: 1. Photosynthesis is an anabolic process: 6 CO2 + 6 H2O + Sunlight ----> C6H12O6 + 6 O2 Carbon Water Sugar Oxygen Dioxide 2. Synthesis of sucrose is an anabolic process: Glucose + Fructose + Energy -------> Sucrose + H2O Monosaccharides Disaccharide V. ATP: Shuttles Chemical Energy in the Cell Coupled Reactions: Endergonic and exergonic reactions are often coupled to each other in living organisms. The energy released by exergonic reactions is used to fuel endergonic reactions. ATP “shuttles” energy around the cell from exergonic reactions to endergonic reactions. One cell makes and hydrolyzes about 10 million ATPs/second. Cells contain a small supply of ATP molecules (1-5 seconds). ATP powers nearly all forms of cellular work: 1. Mechanical work: Muscle contraction, beating of flagella and cilia, cell movement, movement of organelles, cell division. 2. Transport work: Moving things in & out of cells. 3. Chemical work: All endergonic reactions. A. Structure of ATP (Adenosine triphosphate) Adenine: Nitrogenous base. Ribose: Pentose sugar, same ribose of RNA. Three Phosphate groups: High energy bonds. B. ATP Releases Energy When Phosphates Are Removed: Phosphate bonds are rich in chemical energy and easily broken by hydrolysis: ATP + H2O ----> ADP + Energy + Pi ADP + H2O ----> AMP + Energy + Pi Structure and Hydrolysis of ATP C. Regeneration of ATP: ATP can be regenerated through dehydration synthesis: ADP + Energy + Pi ----> ATP + H2O Phosphorylation: Transfer of a phosphate group to a molecule. Requires energy. The energy required for this endergonic reaction is obtained by trapping energy released by other exergonic reactions (E.g.: Cellular respiration). ATP Shuttles Energy From Exergonic Reactions to Endergonic Reactions VI. Enzymes: Protein molecules that catalyze the reactions of living organisms. Enzymes increase the rate of a chemical reaction without being consumed in the process. Name: Substrate (or activity) + ase suffix Examples: Sucrase Lipase Proteinase Dehydrogenase (Removes H atoms) Enzymes are specific: Catalyze one or a few related reactions. Enzymes are efficient. Can increase the rate of a reaction 10 to billions of times!!!! VI. Enzymes: Enzymes increase the rate of a chemical reaction by lowering the activation energy required to initiate the reaction. Activation energy of a reaction: Energetic barrier that reactant molecules must overcome for reaction to proceed. Creation of new bonds requires breaking of old bonds. Both exergonic and endergonic reactions Transition state :“Intermediate” state of reactants Enzymes Lower the Energy of Activation of a Chemical Reaction Enzyme Mechanism of Action: 1. Binding: Enzyme binds to the reactant(s), forming an enzyme-substrate complex. Substrate: The reactant the enzyme acts upon to lower the activation energy of the reaction. Active site: Region on enzyme where binding to substrate occurs. • Active site dependent upon proper 3-D conformation. Enzyme Mechanism of Action: 2. Induced fit model: After enzyme binds to substrate, it changes shape and lowers activation energy of the reaction by one of several mechanisms: Straining chemical bonds of the substrate Bringing two or more reactants close together Providing “micro-environment” conducive to reaction 3. Release: Once product is made, it is released from active site of enzyme. Enzyme is ready to bind to another substrate molecule. Mechanism of Enzyme Action VII. Environmental Factors Affect Enzyme Function A. Temperature: Optimal temperature. Most reactions are too slow at low temperatures. o Most enzymes are denatured over 50-60 C. B. Salt concentration: Optimal concentrations vary for each enzyme. C. pH: Optimal pH varies, but most enzymes work best close to pH 7. Pepsin optimal pH is 2 Amylase optimal pH is 8.5 D. Other essential molecules are required by some enzymes: Cofactors: Inorganic atoms that must bind (Cu, Fe, Zn) Coenzymes: Organic compounds that must bind (vitamins) VII. Regulation of enzyme activity A. Concentration of substrate (enzyme constant) B. Concentration of enzyme (substrate constant) C. Enzyme Inhibitors Competitive inhibitors: Bind to active site of enzyme. Inhibitor resembles normal substrate. Example: • AZT: An AIDS drug. Inhibits an HIV enzyme responsible for viral replication. Resembles nucleotide. Noncompetitive inhibitors: Bind to another site (allosteric site) of enzyme. Active site is altered and unable to bind substrate. Inhibitor usually does not resemble substrate. Competitive versus Noncompetitive Enzyme Inhibition VII. Regulation of enzyme activity D. Feedback Inhibition: Final product of a metabolic pathway can inhibit enzymes that catalyze its synthesis. Enzyme 1 Enzyme 2 Enzyme 3 Enzyme 4 A ---------> B ---------> C ----------> D --------> E (Product) Product (E) inhibits enzyme 1, shutting down pathway. Example: ATP regulates its own production this way. VII. Regulation of enzyme activity E. Inhibition can be temporary or permanent: Reversible inhibition: Inhibitor binds through weak bonds (H-bonds). Inhibitor is released when more
Details
-
File Typepdf
-
Upload Time-
-
Content LanguagesEnglish
-
Upload UserAnonymous/Not logged-in
-
File Pages60 Page
-
File Size-