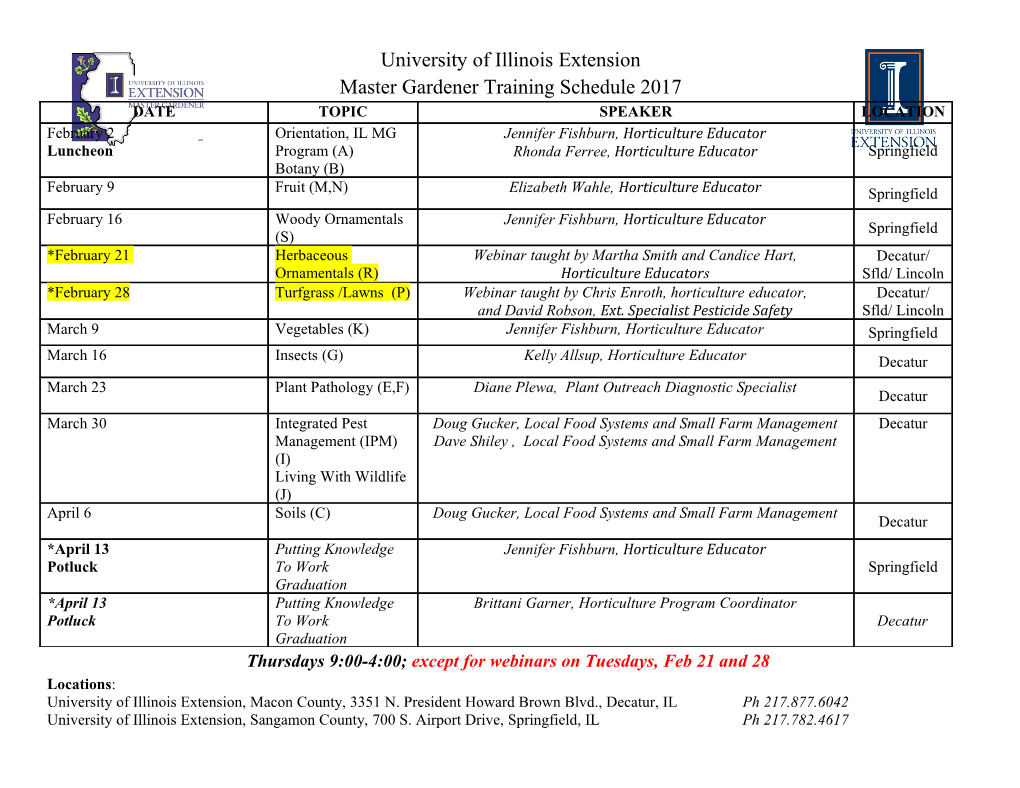
Surface and magnetic polaritons on two-dimensional nanoslab-aligned multilayer structure Zhijian Zhang,1 Keunhan Park,2 and Bong Jae Lee3,∗ 1Department of Mechanical Engineering and Material Science, University of Pittsburgh, Pittsburgh, Pennsylvania 15261, USA 2Department of Mechanical, Industrial and Systems Engineering, University of Rhode Island, Kingston, Rhode Island 02881, USA 3 Department of Mechanical Engineering, Korea Advanced Institute of Science and Technology, Daejeon 305-701, South Korea ∗[email protected] Abstract: The present study theoretically investigates the radiative prop- erties of a two-dimensional (2-D) multilayer structure that has a dielectric spacer between a metallic substrate and square cross-sectional metallic gratings. Differently from the one-dimensional metallic strips coated on a dielectric spacer atop an opaque metallic film [Opt. Express 16, 11328 (2008)], the 2-D metallic gratings can support the localized surface plasmon in addition to the propagating surface plasmon along the metal-dielectric interface. Moreover, the presence of a dielectric spacer also allows the ex- citation of magnetic polaritons. Underlying mechanisms of the surface and magnetic polartions on the proposed structure are elucidated by employing the 2-D rigorous coupled-wave analysis. The results obtained in this study will advance our fundamental understanding of light-matter interaction at the nanometer scale and will facilitate the development of engineered nanostructures for real-world applications, such as thermophotovoltaic and photovoltaic devices. © 2011 Optical Society of America OCIS codes: (160.4760) Optical properties; (240.5420) Polaritons. References and links 1. J. N. Anker, W. P. Hall, O. Lyandres, N. C. Shah, J. Zhao, and R. P. Van Duyne, “Biosensing with plasmonic nanosensors,” Nat. Mater. 7, 442–453 (2008). 2. S. Pillai, K. R. Catchpole, T. Trupke, and M. A. Green, “Surface plasmon enhanced silicon solar cells,” J. Appl. Phys. 101, 093105 (2007). 3. W. Srituravanich, N. Fang, C. Sun, Q. Luo, and X. Zhang, “Plasmonic nanolithography,” Nano Lett. 4(6), 1085– 1088 (2004). 4. I. R. Hooper and J. R. Sambles “Surface plasmon polaritons on thin-slab metal gratings,” Phys. Rev. B 67, 235404 (2003). 5. H. F. Ghaemi, T. Thio, and D. E. Grupp, “Surface plasmons enhance optical transmission through subwavelength holes,” Phys. Rev. B 58, 6779–6782(1998). 6. T. Thio, K. M. Pellerin, R. A. Linke, H. J. Lezec, and T. W. Ebbesen, “Enhanced light transmission through a single subwavelength aperture,” Opt. Lett. 26(24), 1972–1974 (2001). 7. C. Zhao and J. Zhang, “Binary plasmonics: launching surface plasmon polaritons to a desired pattern,” Opt. Lett. 34(16), 2417–2419 (2009). 8. S. Chang and S. K. Gray, “Surface plasmon generation and light transmission by isolated nanoholes and arrays of nanoholes in thin metal films,” Opt. Express 13(8), 3150-3165 (2008). #149604 - $15.00 USD Received 21 Jun 2011; revised 30 Jul 2011; accepted 3 Aug 2011; published 10 Aug 2011 (C) 2011 OSA 15 August 2011 / Vol. 19, No. 17 / OPTICS EXPRESS 16375 9. J. W. Lee, M. A. Seo, D. H. Kang, K. S. Khim, S. C. Jeoung, and D. S. Kim, “Terahertz electromagnetic wave transmission through random arrays of single rectangular holes and slits in thin metallic sheets,” Phys. Rev. Lett. 99, 137401 (2007). 10. M. A. Seo, A. J. L. Adam, J. H. Kang, J. W. Lee, K. J. Ahn, Q. H. Park, P. C. M. Planken, and D. S. Kim, “Near field imaging of terahertz focusing onto rectangular apertures,” Opt. Express 16(23), 20484–20489 (2008). 11. M. Yamamoto, K. Araya, and F. J. Garcia de Abajo, “Photon emission from silver particles induced by a high- energy electron beam,” Phys. Rev. B 64, 205419 (2001). 12. A. J. Haes and R. P. Van Duyne, “A nanoscale optical biosensor: sensitivity and selectivity of an approach based on the localized surface plasmon resonance spectroscopy of triangular silver nanoparticles,” J. Am. Chem. Soc. 124(35), 10596–10640 (2002). 13. J. M. Pitark, V. M. Silkin, E. V. Chulkov, and P. M. Echenique, “Theory of surface plasmons and surface-plasmon polaritons,” Rep. Prog. Phys. 70, 1–87 (2007). 14. H. Raether, Surface Plasmons on Smooth and Rough Surfaces and on Gratings (Springer-Verlag, 1988). 15. A. K. Sarychev, G. Shvets, and V. M. Shalaev, “Magnetic polariton resonance,” Phys. Rev. E 73, 036609 (2006). 16. J. B. Pendry, A. J. Holden, D. J. Robbins, and W. J. Stewart, “Magnetism from conductors and enhanced nonlinear phenomena,” IEEE Trans. Microwave Theory Tech. 47, 2075–2084 (1999). 17. S. Linden, C. Enkrich, M. Wegener, J. F. Zhou, T. Koschny, and C. M. Soukoulis, “Magnetic response of meta- materials at 100 Terahertz,” Science 306, 1351–1353 (2004). 18. C. Enkrich, M. Wegener, S. Linden, S. Burger, L. Zschiedrich, F. Schmidt, J.F. Zhou, T. Koschny, and C. M. Soukoulis, “Magnetic metamaterials at telecommunication and visible frequencies,” Phys. Rev. Lett. 95, 203901 (2005). 19. J. F. Zhou, E. N. Economon, T. Koschny, and C. M. Soukoulis, “Unifying approach to left-handed material design,” Opt. Lett. 31(24), 3620–3622 (2006). 20. M. Kafesaki, I. Tsiapa, N. Katsarakis, T. Koschny, C. M. Soukoulis, and E. N. Economou, “Left-handed meta- materials: the fishnet structure and its variations,” Phys. Rev. B 75, 2345114 (2007). 21. J. Valentine, S. Zhang, T. Zentgraf, E. Ulin-Avila, D. A. Genov, G. Bartal, and X. Zhang, “Three-dimensional optical metamaterial with a negative refractive index,” Nature 455, 376–379 (2008). 22. B. J. Lee, L. P. Wang, and Z. M. Zhang, “Coherent thermal emission by excitation of magnetic polaritons between periodic strips and a metallic film,” Opt. Express 16(15), 11328–11336 (2008). 23. W. Cai and V. Shalaev, Optical Metamaterials: Fundamentals and Applications (Springer, 2009). 24. E. D. Palik, Handbook of Optical Constants of Solids (Academic Press, 1998). 25. B. J. Lee, Y.-B. Chen, and Z. M. Zhang, “Confinement of infrared radiation to nanometer scales through metallic slit arrays,” J. Quant. Spectrosc. Radiat. Trans. 109, 608–619 (2008). 26. B. J. Lee, Y.-B. Chen, and Z.M. Zhang, “Transmission enhancement through nanoscale metallic slit arrays from the visible to mid-infrared,” J. Comput. Theor. Nanosci. 5(2), 201–213 (2008). 27. M. G. Moharam and T. K. Gaylord, “Rigorous coupled-wave analysis of planar-grating diffraction,” J. Opt. Soc. Am. 71(7), 811–818 (1981). 28. M. G. Moharam and T. K. Gaylord, “Rigorous coupled-wave analysis of metallic surface-relief gratings,” J. Opt. Soc. Am. A 3(11), 1780–1789 (1986). 29. Y.-B. Chen and K.-H. Tan, “The profile optimization of periodic nano-structure for wavelength-selective ther- mophotovoltaic emitters,” Int. J. Heat Mass Transfer 53, 5542–5551 (2010). 30. J. Jiang, Rigorous Analysis and Design of Diffractive Optical Elements (Ph. D. Dissertation, The University of Alabama in Huntsville, 2000). 31. J. D. Jackson, Classical Electrodynamics (John Wiley & Sons, 1999). 32. V. A. Podolskiy, A. K. Sarychev, and V. M. Shalaev, “Plasmon modes in metal nanowires and left-handed mate- rials,” J. Nonlinear Opt. Phys. Mater. 11, 65–74 (2002). 33. K. Park, B. J Lee, C. Fu, and Z. M. Zhang, “Study of the surface and bulk polaritons with a negative index metamaterial,” J. Opt. Soc. Am. B 22(5), 1016–1023 (2005). 34. C. F. Bohren and D. R. Huffman, Absorption and Scattering of Light by Small Particles (Wiley-VCH, 1998). 35. E. Hutter and J. H. Fendler, “Exploitation of Localized Surface Plasmon Resonance,” Adv. Mat. 16(19), 1685– 1706 (2004). 36. M. A. El-Sayed,“Some interesting properties of metals confined in time and nanometer space of different shapes,” Acc. Chem. Res. 34(4), 257–264 (2001). 1. Introduction Tailoring the radiative properties, such as reflectance, transmittance, and absorptance, with en- gineered nanostructures has recently drawn much attention due to their great potential in many promising applications, such as biosensing [1], thermophotovoltaic and photovoltaic energy conversions [2], and nano-manufacturing [3]. The spectral- and/or directional-selectivity in the #149604 - $15.00 USD Received 21 Jun 2011; revised 30 Jul 2011; accepted 3 Aug 2011; published 10 Aug 2011 (C) 2011 OSA 15 August 2011 / Vol. 19, No. 17 / OPTICS EXPRESS 16376 radiative properties of engineered nanostructures often result from the excitation of resonance phenomena, such as surface plasmon polariton (SPP). Note that SPPs can be observed in pe- riodic metallic structures, such as one-dimensional (1-D) gratings [4], two-dimensional (2-D) hole arrays [5], bulls eye structure [6], and binary plasmonic structure [7], where the reciprocal lattice vector of the periodic structure compensates the momentum difference between the sur- face plasmon and the incident light. One the other hand, the localized surface plasmon (LSP) has been found on the isolated subwavelength structure, such as a single hole on a thin metal film [8–10] and nanoparticles [11, 12]. The key underlying mechanism of the SPP and LSP is the coupling of the incident wave with the collective charge density oscillations on the conduc- tor [13]; hence they are usually observed at the metal-dielectric interface. Since the energy and momentum of the incident light have to match with those of the SPP, the SPP resonance usually depends on the frequency and the incidence angle of the incident wave [14]. In addition to SPPs, the magnetic resonance (or magnetic polariton) has been extensively studied since it can induce the coupling between the magnetic-field component of the incident wave and the matter, allowing one to tailor the optical properties of materials in an unprece- dented manner [15]. For instance, Pendry first proposed to use a split-ring resonator to achieve the effective negative permeability resulting from the diamagnetism [16]. Afterwards, the sin- gle split-ring [17] and U-shape cells [18] have been demonstrated as the magnetic resonators.
Details
-
File Typepdf
-
Upload Time-
-
Content LanguagesEnglish
-
Upload UserAnonymous/Not logged-in
-
File Pages15 Page
-
File Size-