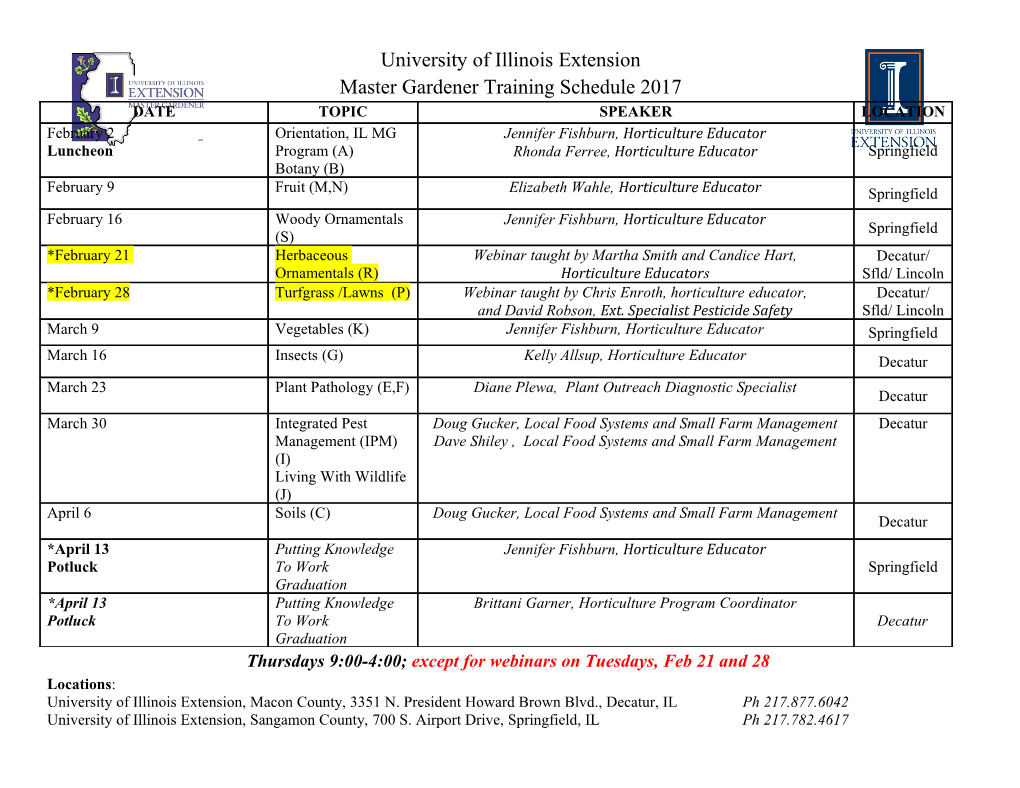
A BRIEF HISTORY OF FRACTOGRAPHY S. P. Lynch+* and S. Moutsos* *Monash University, Clayton Vic. 3168 Australia, + DSTO, Fishermans Bend, Vic. 3207 Australia ABSTRACT Fracture surfaces have, no doubt, been studied throughout the history of mankind, probably starting with observations on stone-age tools. In the 16-18th centuries, the macroscopic appearance of fracture surfaces was used to assess the quality of metallic materials, with studies by Réaumur in 1722 being the most notable. However, it was not until 1943 that fracture surfaces were first examined at high-magnifications (using optical microscopy up to 1,000x), and that the first attempts were made to examine replicas of fracture surfaces using transmission-electron microscopy (TEM). Early replicas had poor fidelity and resolution, and it was not until 1956 that Crussard et al. pioneered high-resolution TEM fractography using shadowed, direct-carbon replicas. This technique (and its subsequent variations) revolutionised fractography and led to a plethora of studies in the 1960’s and 70’s. It therefore seems appropriate to commemorate the 50th anniversary of high-resolution electron fractography with a review of how it, and subsequent scanning electron microscopy (SEM) and other techniques, have led to a better understanding of fracture processes. Such understanding has been invaluable in failure analysis and in developing improved materials. Milestone observations for a number of important modes of fracture in inert environments including cleavage, brittle intergranular fracture, dimpled overload fractures, and fatigue fractures, are described first, followed by examples of key observations for fractures produced in embrittling environments. Overload Fracture by Cleavage Cleavage of minerals had probably been noted in early times, but the first significant scientific observations appear to have been made by Guglielmini (1688) and subsequently by Haüy (1784), who noted that the cleavage always occurred on the same plane, with Haüy producing rhombohedral crystals of calcite by cleavage regardless of the external shape of the crystals [1]. In the sixteenth to eighteenth centuries, numerous examples of the use of fracture-surface appearance to assess both the quality of metals and the effects of alloying elements were reported. Fractures exhibiting smooth and bright planes, i.e. cleavage (or brittle intergranular) facets, were recognized as indicative of poor quality. The most comprehensive of these early fractographic studies was by Réaumur (1722) who described seven classes of iron according to their fracture-surface appearance. The first type exhibited “….very brilliant white platelets, which appear to be as many little mirrors of irregular shape and arrangement…” – a nice description of cleavage in polycrystals (Fig.1) [2, 3] #. In the mid-to-late nineteenth century, observations that large grains of iron alloys could be cleaved to produce small cubes [4] (like the much earlier observations for minerals) strongly supported the case that metals were also crystalline – a view that was then not universally accepted. The first detailed studies of cleavage fractures at high magnifications, showing a wealth of detail, were published in 1943 by Zappfe and Moore [5], and then in 1945 by Zappfe and Clogg [6] who used the term ‘fractography’ for the first time. They showed, for example, that {100} fractures in an iron-silicon alloy exhibited river lines (converging steps parallel to the direction of crack growth), tongues (where twins had formed ahead of cracks with subsequent crack growth along the twin-matrix interface), and slight tilts across sub-grain boundaries (Fig. 2). Hull and Beardmore [7] subsequently showed that river lines on {100} cleavage planes occurred preferentially on <110> directions. Examination of cleavage fracture surfaces using TEM by Crussard and his colleagues [8] in 1956 and subsequently by Beachem [9], Henry and Plateau [10], and others, revealed finer steps and tongues than detected by optical microscopy, and indicated that areas between these features were featureless even at high magnifications (Fig. 3). Recent scanning-tunnelling microscopy (STM) of cleaved chromium single crystals has shown that fractures can be atomically flat between atomic-scale steps (Fig. 4) [11]. The preference for cleavage on {100} planes in <110> directions for bcc metals is somewhat surprising since Griffith’s thermodynamic surface-energy criterion for brittle fracture (1921)[12] predicts that (i) the favoured cleavage plane (for elastically isotropic metals) should be the {110} plane which has the minimum surface energy, and (ii) there should be no preferred direction. Etch pits on cleavage fracture surfaces, and other observations, show that some dislocation activity # These references describe the early history of fractography in detail, show other examples of sketches by Réaumur , and provide original references of early studies. Also note that micrographs from the literature have been cropped to save space, and clearer scale bars and other annotations have been added. accompanies cleavage, and it has been suggested that preferred cleavage planes and directions are those for which the extent of plasticity around cracks is minimised. More recent work [13] suggests that cleavage anisotropy may be intrinsic and associated with ‘lattice-trapping’. For example, the cleavage (decohesion) process could involve incipient (reversible) dislocation emission at crack tips [14], i.e. shear plus tensile displacements of atoms at crack tips rather than just tensile separation of atoms, which would be facilitated if crack fronts were along <110> directions – the line of intersection of crack planes and slip planes. For the {100} plane, there would be two easy <110> directions for cleavage whereas there would be only one such direction for the {110} plane – which might explain why {100} planes are often favoured. Figure 1. Sketches by Réaumur (1722) of cleavage fracture Figure 2. Optical fractography of a {100} cleavage fracture of polycrystalline iron, described as “… little mirrors of surface of an Fe-Si alloy, exhibiting river lines, tongues, and irregular shape and arrangement’’ [2, 3]. sub-grain boundaries (Zappfe and Clogg, 1945) [6]. Figure 3. TEM of replica of fracture surface of a steel (ferrite) Figure 4. STM of {001} fracture surface of Cr single crystal produced by impact at -40ºC showing fine river lines cleaved in vacuum (in situ) at 4.2K showing cubic array of (Crussard et al. 1956) [8]. ‘atoms’ (Kolesnychenko et al., 2001) [11]. Brittle Intergranular Overload Fracture Many of the brittle fractures observed in early times would often have been intergranular rather than cleavage, due to either the presence of low-melting-point impurity phases or the segregation of impurity atoms at grain boundaries. Intergranular fracture to produce single grains of steel from an overheated bar (Tschernoff, 1878)[3] (Fig. 5) provided an appreciation of the complex shape of metal grains for the first time, and the effect of heat-treatment on grain size was determined from fractographic observations by Brinell in 1885 [3]. Phosphorus impurities as a cause of intergranular embrittlement of steels was first mentioned in 1898, but it was not until the development of Auger Electron Spectroscopy in 1969 that it was demonstrated that fractions of a monolayer of impurities at grain boundaries could produce embrittlement, with the degree of embrittlement increasing with increasing levels of segregation [15,16]. The appearance of intergranular fracture surfaces at high magnifications, like other fracture modes, was first characterised by TEM of replicas from 1956 onwards, and numerous examples were illustrated in Henry and Plateau’s classic book “La Microfractographie” in 1967 [10]. Intergranular fracture surfaces resulting from segregation of impurities often appear smooth and featureless (except for particles in some cases), but blocky or wavy steps are sometimes observed since segregated grain boundaries often develop crystallographic facets to minimise the grain-boundary energy (Fig. 6). Embrittlement is generally explained in terms of an atomically brittle ‘decohesion’ process due to segregation-induced weakening of interatomic bonds across grain-boundaries [e.g. 17]. Two-dimensional grain-boundary phase changes dependent on the degree of segregation and temperature may also be involved – a concept first proposed for temper-embrittlement of steels in 1968 [18, 19]. Significant plasticity is sometimes evident on ‘brittle’ intergranular fracture surfaces and, hence, crack growth may occur by a very localised plasticity process rather than (or in addition to) decohesion in some cases [19]. Figure 5. Photograph of a single grain of steel produced by Figure 6. TEM of replicas of brittle intergranular fractures at intergranular fracture of an overheated bar (Tschernoff, -196ºC in (a) Fe 0.018% O, and (b) commercial-purity Ni with S 1878, reproduced in Smith, 1988 [3]). segregation at boundaries (Henry and Plateau, 1968)[10]. Dimpled Overload Fracture Réamur’s 1722 classification of different sorts of fractures included fibrous ones (and mixtures of fibrous and mirror-like ones), and fibrous fractures were recognised as indicative of good quality (tough) material. Radial markings (steps) diverging from the fibrous fracture origins were first noted by Martens in 1887 (Fig. 7) [2] - an important observation widely used in present-day failure analysis. Fibrous ductile fractures were too rough to
Details
-
File Typepdf
-
Upload Time-
-
Content LanguagesEnglish
-
Upload UserAnonymous/Not logged-in
-
File Pages12 Page
-
File Size-