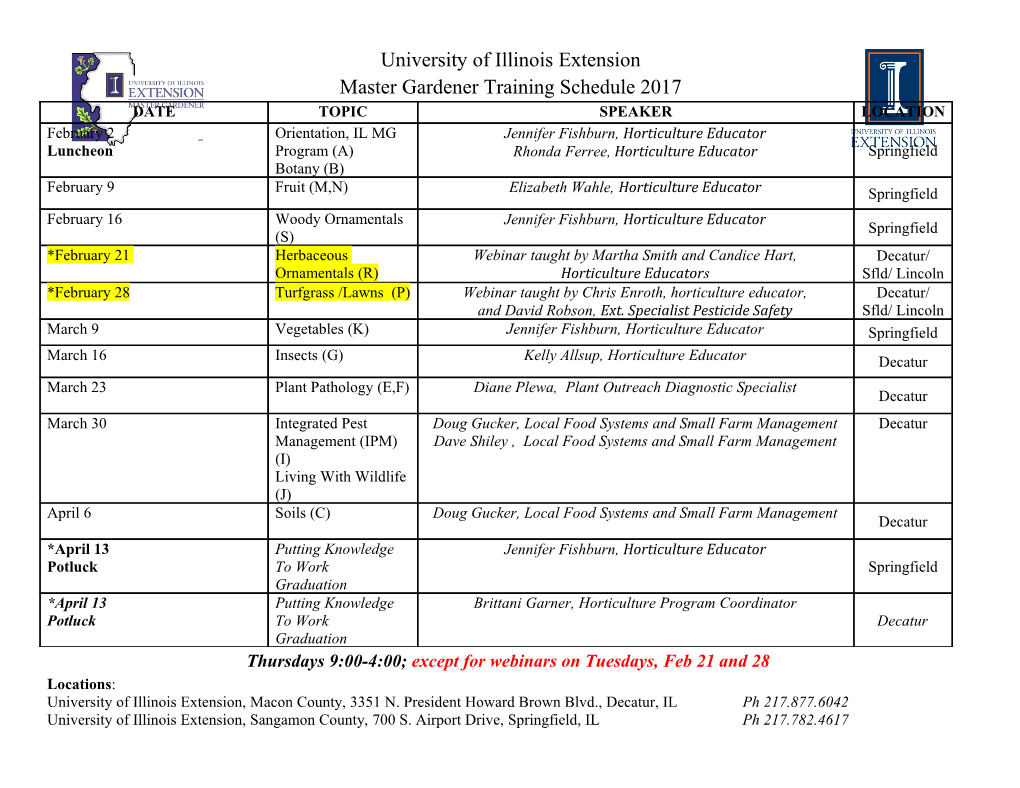
Computers Astronomer Edward Charles Pickering's Harvard “computers" Annie Jump Cannon Classified 200,000 stars in 3 years: OBAFGKMLTY (Average: 2 minutes per star, six days per week) Could eventually classify three stars per minute. Classified 350,000 stars in her lifetime. Obviously, Brilliant Astronomers Feel Glad Knowing Many Luminous Types, Yo Q: When stars are sorted according to spectral type based on the width of the Hydrogen absorption lines what physical property underlies the order? Meet Astronomer Barbie, Available Fall, 2019 Q: When stars are sorted according to spectral type based on the width of the Hydrogen absorption lines what physical property underlies the order? A: Most broadening is due to Doppler shifts caused by bulk motion or nonzero temperature of the emitting regions. Recently, OBAFGKM à OBAFGKMLTY because we can see fainter stars now Obviously, Brilliant Astronomers Feel Glad Knowing Many Luminous Types, Yo Main- Main- Main- Fraction of sequence Effective Vega-relative sequence sequence Hydrogen all main Class Chromaticity mass Temperature chromaticity radius luminosity lines sequence (solar masses) (solar radii) (bolometric) stars O ≥ 30,000 K blue blue ≥ 16 M☉ ≥ 6.6 R☉ ≥ 30,000 L☉ Weak ~0.00003% deep blue B 10,000–30,000 K blue white 2.1–16 M 1.8–6.6 R 25–30,000 L Medium 0.13% white ☉ ☉ ☉ A 7,500–10,000 K white blue white 1.4–2.1 M☉ 1.4–1.8 R☉ 5–25 L☉ Strong 0.6% F 6,000–7,500 K yellow white white 1.04–1.4 M☉ 1.15–1.4 R☉ 1.5–5 L☉ Medium 3% G 5,200–6,000 K yellow yellowish white 0.8–1.04 M☉ 0.96–1.15 R☉ 0.6–1.5 L☉ Weak 7.6% pale yellow K 3,700–5,200 K light orange 0.45–0.8 M 0.7–0.96 R 0.08–0.6 L Very weak 12.1% orange ☉ ☉ ☉ light orange M 2,400–3,700 K orange red 0.08–0.45 M ≤ 0.7 R ≤ 0.08 L Very weak 76.45% red ☉ ☉ ☉ The bolometric magnitude of a star is a measure of the total radiation of a star emitted across all wavelengths of the electromagnetic spectrum. See: Chromaticity: color https://en.wikipedia.org/wiki/Stellar_classification Luminosity is the total amount of electromagnetic energy emitted per unit of time by a star, galaxy, or other astronomical object. (Watts) Boltzman equation: What proportion of electrons are in each energy state? Saha equation: What proportion of atoms are ionized? Hertzsprung and Russell: Giants and dwarfs Absolute Magnitude Smaller total Larger total energy output energy output Hotter Spectral Type Spectral Cooler Radius Q: What goes this way? Mass Radius Q: What is the density? (Thanks, Ken) We’ve got better HR diagrams now! Stars on the main sequence are burning Hydrogen. As stars exhaust their Hydrogen, they move off the main sequence. How they evolve from there depends on their mass. The color of a star gives a measure of its temperature . The human eye only sees part of the blackbody radiation spectrum. The color we see for a star depends on the average only over the visible part. As the temperature increases, the visual effect traces a line across the possible colors. The line misses green entirely, and even at infinite temperature we would only see blue- white. Luminosity Spectra at the same spectral type show variation with size. Denser stars have higher surface gravity. The increased pressure leads to pressure broadening of spectral lines. Thus, the broadening of spectral lines from a giant star is much less than from a dwarf star, even if the stars have the same color (and hence temperature). Therefore, broadening of spectral lines varies with the luminosity. We can estimate both the luminosity and the temperature of a star from its spectrum, and place it on the HR diagram. https://www.youtube.com/watch?v=K2fZxOPWbDU http://hubblesite.org/video/704/news Elizabeth Langdon Williams (February 8, 1879 in Putnam, Connecticut – 1981[1]) was an American human computerand astronomer. She graduated from MIT in physics in 1903 as one of their earliest female graduates,[2] and was hired by Percival Lowell in 1905 to work from an office in Boston.[3] Her role was to perform the mathematical calculations required to predict the location of a proposed Planet X that Lowell hypothesised affected the orbits of the known planets Neptuneand Uranus.[4] She was the head human computer for Lowell in 1915. Her calculations led to predictions for the location of the unknown planet, but Lowell died in 1916 meaning that the project was discontinued. In the late 1920s the project was resumed and Clyde Tombaugh was hired to lead it. Tombaugh used Lowell's predictions to locate an image in a region of the sky photographed in 1915 that he identified as a new planet, named Pluto in 1930.[5] Williams continued to work at Lowell Observatory after Lowell's death, moving from Boston to the observatory itself at Flagstaff in 1919. In 1922, Williams married another astronomer, George Hall Hamilton.[1][6] She was then dismissed from her position at the observatory by Constance Lowell since it was considered inappropriate to employ a married woman.[7]Williams and her husband were subsequently employed at an observatory in Mandeville, Jamaica run by Harvard College Obervatory where they worked together. In 1935, William's husband died. She moved to New Hampshire and subsequently died in poverty. Evolution of a Planetary Nebula formation solar mass star Thermal Pulse Asymptotic Giant Branch Early Asymptotic Giant Branch Red Giant Branch Sub-Giant Branch Hydrogen fusion in core Zero Age Main Sequence 90 of stellar lifetime Evolution of a Planetary Nebula formation solar mass star Thermal Pulse Asymptotic Giant Branch Early Asymptotic Giant Branch Red Giant Branch Sub-Giant Branch Hydrogen fusion in core Zero Age Main Sequence 90 of stellar lifetime Evolution from the main sequence: Low (∼ 1 M⊙) and intermediate (∼ 5 M⊙ – 8 M⊙ ) mass stars • As Hydrogen fuses to Helium the proportion of Helium to Hydrogen in the core increases, but not enough to fuse Helium. The temperature decreases à outer layers of the star compress the core à the temperature of the core increases enough to support the star The average density of the core increases. • Cessation of Hydrogen burning in the core Hydrogen fusion ceases in the core, continuing in a Hydrogen burning shell. The Helium core is nearly constant temperature, with increasing density toward the center. • Increased temperature drives Hydrogen fusion faster • Luminosity of the star increases • The outer layers of the star force expand Evolution from the main sequence: Low (∼ 1 M⊙) and intermediate (∼ 5 M⊙ – 8 M⊙ ) mass stars • The Subgiant Branch At the Schönberg-Chandrasekhar Limit the isothermal Helium core cannot support the outer layers of the star. The core is compressed, heaLng the Hydrogen burning layer. Luminosity increases rapidly, driving the outer layers of the star outward. David Taylor, Evanston, IL The star moves to the right in the Hertzsprung-Russell diagram. This is the subgiant branch. Evolution from the main sequence: Low (∼ 1 M⊙) and intermediate (∼ 5 M⊙ – 8 M⊙ ) mass stars Planetary Nebula formation Thermal Pulse Asymptotic Giant Branch • The Red Giant Branch Early Asymptotic The star develops convection Giant Branch currents that mix deeper and deeper layers of the star with the Red Giant surface layers. This increases energy Branch transport; the star greatly increases in size and magnitude. This “first dredge up” brings Sub-Giant Branch processed elements from the core out to the surface, providing an important spectroscopic test of the predictions of stellar models. Zero Age Main Sequence Evolution from the main sequence: Low (∼ 1 M⊙) and intermediate (∼ 5 M⊙ – 8 M⊙ ) mass stars • Triple alpha fusion The triple alpha process begins almost explosively, releasing 1011 mes the luminosity of the sun. The burst lasts only a few seconds and most of the energy is absorbed by the outer layers of the star. • Helium burns into Carbon and Oxygen At the top of the red giant branch, the core temperature and density become high enough for quantum tunneling between alpha particles. Helium nuclei can fuse with another alpha particle to produce Beryllium, 8Be. This unstable and decay backs to Helium unless, within the lifetime of the Beryllium, a third alpha particle fuses to form Carbon, 12C. This is the triple alpha process. An additional fusion of an alpha particle with the Carbon can produce Oxygen. Evolution from the main sequence: Low (∼ 1 M⊙) and intermediate (∼ 5 M⊙ – 8 M⊙ ) mass stars Bluer • The Horizontal Branch Helium fusion conLnues at the core of the star. The burning proceeds much faster than the main sequence Hydrogen fusion. The star contracts and moves to the leW Horizontal branch (blueward) along the horizontal branch, and may pulse periodically. As the Helium is converted into Carbon and Oxygen, the Schönberg-Chandrasekhar limit is again approached at the core. The CO core contracts, a zone of Helium outside the core forms and conLnues burning, driving the outer layers to expand and cool. Evolution from the main sequence: Low (∼ 1 M⊙) and intermediate (∼ 5 M⊙ – 8 M⊙ ) mass stars Planetary Nebula formation Thermal Pulse Asymptotic Giant Branch • Early Asymptotic Early Asymptotic Giant Giant Branch Branch A burning Helium shell now Red Giant drives expansion. Branch • The core temperature reaches 2×108K. The outer layers absorb much of the energy, expanding and Sub-Giant Branch cooling. • Convection begins again and deepens, giving the second dredge up. Zero Age • The temperature of the star Main Sequence continues to rise, driving it higher in the Hertzsprung-Russell diagram Evolution from the main sequence: Low (∼ 1 M⊙) and intermediate (∼ 5 M⊙ – 8 M⊙ ) mass stars Planetary Nebula • Thermal-Pulse AsymptoCc Giant Branch formation As the star grows ho\er the hydrogen shell Thermal Pulse reignites and dominates the star’s luminosity.
Details
-
File Typepdf
-
Upload Time-
-
Content LanguagesEnglish
-
Upload UserAnonymous/Not logged-in
-
File Pages35 Page
-
File Size-