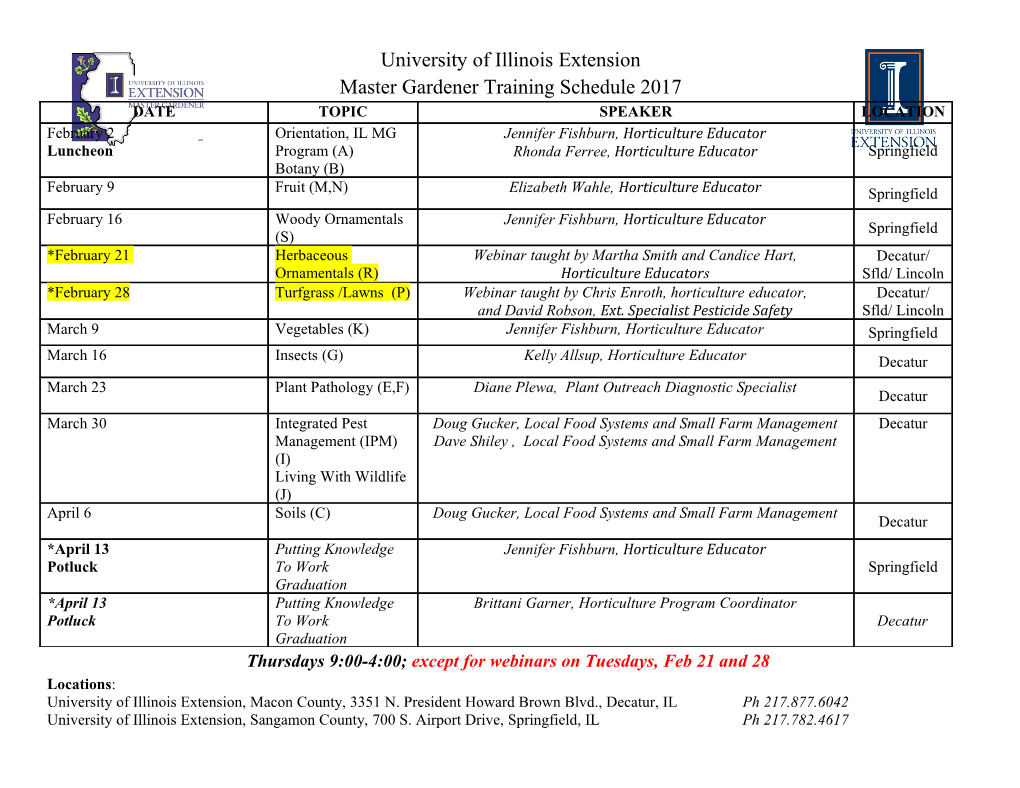
Modelling the Hydrodynamic Drag Force of Flexible Riparian Woodland Peter Whittaker Hydro-environmental Research Centre Cardiff University A thesis submitted for the degree of Doctor of Philosophy March 2014 "We must never cease from exploration. And the end of all our exploration will be to arrive where we began and to know the place for the first time." T. S. Eliot i Acknowledgements I would like to thank Dr. C. Wilson and Prof. R.A. Falconer for their counselling and guidance as my supervisors. Additionally, this thesis would not have been possible without the generous funding from Forest Research and the assistance of H. Thomas in conducting field work. I am also grateful to my colleagues in the HRC for provoking stimulating discussion and providing many sanity preserving distrac- tions. Finally, I would like to thank my family and Vaiva for all their support, encouragement and interest throughout my studies. iii Declaration This work has not been submitted in substance for any other degree or award at this or any other university or place of learning, nor is being submitted concurrently in candidature for any degree or other award. Signed . Date . Statement 1 This thesis is being submitted in partial fulfilment of the requirements for the degree of PhD. Signed . Date . Statement 2 This thesis is the result of my own independent work / investigation, except where otherwise stated. Other sources are acknowledged by explicit references. The views expressed are my own. Signed . Date . Statement 3 I hereby give consent for my thesis, if accepted, to be available for photocopying and for inter-library loan, and for the title and summary to be made available to outside organisations. Signed . Date . Statement 4 I hereby give consent for my thesis, if accepted, to be available for photocopying and for inter-library loans after expiry of a bar on access previously approved by the Academic Standards & Quality Committee. Signed . Date . v Abstract In this thesis, two practical models for predicting the drag force exerted on flexible riparian vegetation under hydrodynamic loading have been developed. The models were formulated based on the results of a unique experimental data set that consisted of high resolution force-velocity and physical property measurements for twenty-one full-scale riparian trees, in both foliated and defoliated conditions. One of the models has then been used to numerically simulate the impact of riparian woodland on the flooding characteristics of a mid-catchment river site. Analysis of photographs and video footage of the trees from the experimental study during drag force testing allowed the frontal projected area to be determined, both in still air and as a function of flow velocity. The observed reductions in projected area and drag coefficient with velocity were normalized using the projected area in still air to provide an empirical relationship between the ‘rigid’ drag coefficient and area Reynolds number. The resulting drag force predictions were found to be accurate when properly calibrated against the vegetation under consideration. A second, more physically based model to predict the reconfiguration of flexible vegetation has been developed based on dimensional analysis of the relevant parameters, including flexural rigidity. The model utilizes a novel vegetative Cauchy number to determine the extent of the reconfiguration and has been shown to be more accurate than two existing drag force models. The model has also been validated against independent drag force data, demonstrating that it is applicable to vegetation of differing scale, morphology and flexibility. Serial and parallel optimizations of an existing two-dimensional hydrodynamic modelling code have enabled detailed numerical simulations of extreme flood events to be undertaken for a mid-catchment river site in Somerset, UK. The results indicated that riparian vegetation has a minimal impact on the downstream flooding characteristics, at least for the small site investigated herein. Significant reductions in key flow properties, namely velocity and bed shear stress, were however observed within the vegetated areas. vii Contents Abstract vii Contents ix List of Figures xiii List of Tables xxi List of Symbols xxvii 1 Introduction 1 1.1 Context and Perspective . 1 1.1.1 Flood Management . 2 1.1.2 Riparian Woodland . 4 1.2 Motivation . 6 1.2.1 Current Practices and Limitations . 6 1.3 Thesis Scope and Objectives . 7 1.3.1 Thesis Layout . 8 2 Literature Review 11 2.1 Introduction . 11 2.2 Drag Force Theory . 11 2.2.1 Drag Coefficient . 13 2.3 Vegetative Drag and Resistance . 14 2.3.1 Idealized Vegetation . 15 2.3.2 Flexible Vegetation . 17 ix Contents 2.3.3 Numerical Modelling Studies . 23 2.4 Summary . 26 3 Experimental Data and Analysis 29 3.1 Introduction . 29 3.2 Experimental Data . 30 3.2.1 Hydralab Experiments . 30 3.2.2 LWI Experiments . 42 3.3 Drag Force Analysis . 44 3.3.1 Impact of Foliage . 45 3.3.2 Reconfiguration . 47 3.4 Projected Area Analysis . 57 3.4.1 In Still Air . 57 3.4.2 Variation with Towing Velocity . 67 3.5 Drag Coefficient Analysis . 73 3.5.1 Foliated . 74 3.5.2 Defoliated . 75 3.5.3 Impact of foliage . 75 3.6 Summary . 77 4 Drag Force Models for Flexible Vegetation 79 4.1 Introduction . 79 4.2 Drag Coefficient Model . 79 4.2.1 Theory . 80 4.2.2 Results and Discussion . 82 4.3 Cauchy Reconfiguration Model . 87 4.3.1 Theory . 87 4.3.2 Results and Discussion . 91 4.3.3 Comparison to Existing Models . 94 4.3.4 Model Parameter Sensitivity Analysis . 99 4.4 Summary . 102 5 Numerical Modelling of Riparian Woodland 105 5.1 Introduction . 105 x Contents 5.2 Governing Equations . 106 5.2.1 Navier-Stokes Equations . 106 5.2.2 Shallow Water Equations . 109 5.3 Numerical Model . 110 5.3.1 DIVAST-TVD . 112 5.3.2 Bed Shear Stress . 113 5.3.3 Vegetative Drag Force . 114 5.3.4 Turbulence Model . 115 5.4 Model Optimization . 116 5.4.1 CPU Optimization . 118 5.4.2 GPU Optimization . 122 5.5 Case Study: Lopen Brook . 126 5.5.1 Digital Elevation Model . 131 5.5.2 Calibration . 134 5.5.3 Boundary Conditions . 138 5.6 Results & Discussion . 139 5.6.1 Scenario 1: No Woodland . 139 5.6.2 Scenario 2: Existing Woodland . 144 5.6.3 Scenario 3: Short Rotation Coppice . 149 5.6.4 Scenario 4: Woodland Expansion . 152 5.6.5 Scenario 5: Floodplain Storage . 155 6 Conclusions and Future Research 161 6.1 Conclusions . 161 6.1.1 Experimental Data and Analysis . 161 6.1.2 Drag Force Models for Flexible Vegetation . 162 6.1.3 Numerical Modelling of Riparian Woodland . 163 6.2 Future Research . 165 References 167 A Vegetation Data 183 B Lopen Brook 187 xi List of Figures 1.1 Aerial photograph of Tewkesbury Abbey taken during the July 2007 flooding of the Avon and Severn rivers. Reproduced from Pitt (2008). 3 2.1 Streamlines for: (a) streamlined object; (b) bluff object. Reproduced from Douglas et al. (2005, pp. 398). 12 2.2 Stagnation pressure for flow around: (a) an idealized plate; (b) a real plate. 13 2.3 Variation in drag coefficient with Reynolds number for two-dimensional objects. Reproduced from Massey (2006, pp. 330). 14 2.4 Comparison of finite difference grid resolutions for: (a) stem-scale; and (b) reach-scale models. Individual plants or stems are represented as circles. 23 3.1 Plan view schematic of the towing carriage at the CEHIPAR facilities. 36 3.2 Component forces against time for a single run incorporating a num- ber of velocities. Reproduced from Xavier (2009). 38 3.3 Variation in drag force with towing velocity for the: (a) foliated; and (b) defoliated A. glutinosa trees from the Hydralab experiments. 39 3.4 Variation in drag force with towing velocity for the: (a) foliated; and (b) defoliated P. nigra trees from the Hydralab experiments. 40 3.5 Variation in drag force with towing velocity for the: (a) foliated; and (b) defoliated S. alba trees from the Hydralab experiments. 41 3.6 Photographs of the fully submerged natural and artificial branches used in the LWI experiments. The images were captured in still water using a submersible digital camera. 42 xiii List of Figures 3.7 Variation in drag force with flow velocity for the: (a) partially sub- merged; and (b) fully submerged branches from the LWI experiments. The natural and artificial plants are denoted using (N) or (A), respec- tively. 43 3.8 Proportion of total drag force caused by foliage for the: (a) A. gluti- nosa; (b) P. nigra; and (c) S. alba Hydralab trees. 46 3.9 Variation in characteristic drag coefficient with velocity for the: (a,b) A. glutinosa; (c,d) P. nigra; and (e,f) S. alba Hydralab trees. The foli- ated and defoliated trees are in the left and right columns, respectively. 48 3.10 Variation in characteristic drag coefficient with velocity for the: (a) partially; and (b) fully submerged LWI branches. 49 3.11 Individual and species-averaged Vogel exponents obtained from a power-law regression on the full force-velocity data sets for the A. glutinosa (A), P. nigra (P) and S. alba (S) Hydralab trees. 52 3.12 One-sided stem area per quartile for the A. glutinosa (A), P. nigra (P) and S. alba (S) Hydralab trees. Error bars show minimum and maximum values depending on pixel area definition. 60 3.13 Minimum, maximum, and average of the north and south projected areas in still air for the foliated A. glutinosa (A), P. nigra (P) and S.
Details
-
File Typepdf
-
Upload Time-
-
Content LanguagesEnglish
-
Upload UserAnonymous/Not logged-in
-
File Pages223 Page
-
File Size-