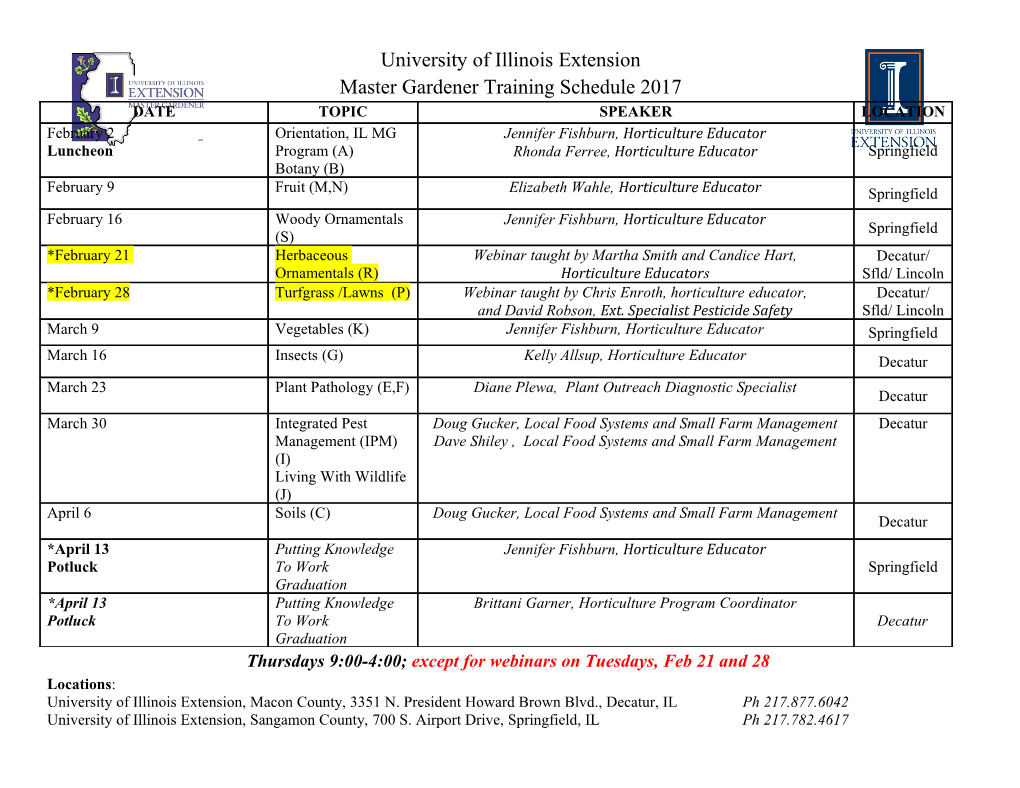
10 7 General vector spaces Proposition & Definition 7.14. Monomial basis of n(R) P Letn N 0. The particular polynomialsm 0,m 1,...,m n n(R) defined by ∈ ∈P n 1 n m0(x) = 1,m 1(x) = x, . ,m n 1(x) =x − ,m n(x) =x for allx R − ∈ are called monomials. The family =(m 0,m 1,...,m n) forms a basis of n(R) B P and is called the monomial basis. Hence dim( n(R)) =n+1. P Corollary 7.15. The method of equating the coefficients Letp andq be two real polynomials with degreen N, which means ∈ n n p(x) =a nx +...+a 1x+a 0 andq(x) =b nx +...+b 1x+b 0 for some coefficientsa n, . , a1, a0, bn, . , b1, b0 R. ∈ If we have the equalityp=q, which means n n anx +...+a 1x+a 0 =b nx +...+b 1x+b 0, (7.3) for allx R, then we can concludea n =b n, . , a1 =b 1 anda 0 =b 0. ∈ VL18 ↓ Remark: Since dim( n(R)) =n+1 and we have the inclusions P 0(R) 1(R) 2(R) (R) (R), P ⊂P ⊂P ⊂···⊂P ⊂F we conclude that dim( (R)) and dim( (R)) cannot befinite natural numbers. Sym- P F bolically, we write dim( (R)) = in such a case. P ∞ 7.4 Coordinates with respect to a basis 11 7.4 Coordinates with respect to a basis 7.4.1 Basis implies coordinates Again, we deal with the caseF=R andF=C simultaneously. Therefore, letV be an F-vector space with the two operations+ and . Let alson := dim(V)< and choose · ∞ a basis =(b 1,...,b n) ofV. B Since is a generating system and linearly independent, eachv fromV has a linear B combination v=α 1b1 +...+α nbn (7.4) where the coefficientsα 1,...,α n F are uniquely determined. We call these numbers ∈ the coordinates ofv with respect to the basis and sometimes writev B for the vector B consisting of these numbers: n A vectorv inV and its coordinate vectorv B inF α1 . n v=α 1b1 +...+α nbn V v B = . F . (7.5) ∈ ←→ . ∈ αn Whenfixing a basis inV , then each vectorv V uniquely determines a coordinate n B ∈ vectorv B F – and vice versa. ∈ Forming the coordinate vector is a linear map The translation of a vectorv V into the n ∈ coordinate vectorv B F defines a linear ∈ map: vector spaceV n Φ :V F ,Φ (v)=v B B → B 1 Φ Φ− More concretely: B � B Φ (α1b1 +...+α nbn) =α 1e1 +...+α nen B vector� space F n For allx,y V andλ F, the mapΦ sat- ∈ ∈ isfies two properties: Φ (x+y)=Φ (x) +Φ (y) (+) B B B Φ (λx) =λΦ (x) ( ) B B · α1 . n v=α 1b1 +...+α nbn V Φ (v)= . F . (7.6) ∈ ←→ B ∈ αn The linear mapΦ is called the basis isomorphism with respect to the basis and B B is completely defined byΦ(b j) =e j forj=1, . , n. 12 7 General vector spaces Example 7.16. An abstract vector is represented by numbers The three functions sin, cos and arctan from the vector space (R), cf. Example 7.4, F span a subspace: V := Span(sin, cos, arctan) (R). ⊂F In the same manner as before, we can show that the three functions are linearly independent. Hence, they form a basis ofV: := (sin, cos, arctan). B Now, if we look at another functionv V given by ∈ v(x) = 8 cos(x) + 15 arctan(x) for allx R, hencev=8 cos + 15arctan. ∈ Then: v=8 cos + 15arctan arctan sin cos Wefind v=8 cos + 15arctan directly by using its coordinates: 0 Φ (v)= 8 . B �15� Rule of thumb:V is completely represented byF n EachF-vector spaceV withn := dim(V)< is represented byF n if youfix a basis =(b ,...,b ) ∞ B 1 n For each vectorv V , there is exactly one coordinate vectorΦ (v) F n. Instead ∈ B ∈ α1 . n of usingv V , one can also do calculations withΦ (v)= . F . ∈ B ∈ αn 7.4 Coordinates with respect to a basis 13 3 2 Example 7.17. The polynomialsp,q 3(R) given byp(x) = 2x x + 7 andq(x) = 2 ∈P − x +3 can be represented with the monomial basis =(m 0,m 1,m 2,m 3) by the coordinate B vectors: 1 2 2 2 Example 7.18. The matrixA= R × has the following coordinate vector with 0 3 ∈ respect to the basis from equation ( 7.1): B � � 1 Φ (A) = 2 . B �3� 3 The matrix3A has the coordinate vector 6 . �9� The matrix 5 0 5 C= 0 2 0 5 0 5 7.4.2 Change of basis For a given basis =(b 1,...,b n) of the vector spaceV , we have the linear map B n Φ :V F ,Φ (bj) =e j for allj B → B 14 7 General vector spaces which is also invertible. We have called it the basis isomorphism. For a given element v=α 1b1 +...+α nbn, we can write: 1 1 1 v=α 1b1 +...+α nbn =α 1Φ− (e1) +...+α nΦ− (en) =Φ − (Φ (v)). B B B B 1 n 1 Φ− :F V,Φ − (ej) =b j for allj B → B Example 7.19. Consider the already introduced monomial basis =(m 2,m 1,m 0) = 2 B (x x , x x, x 1) of the space 2(R) and the polynomialp 2(R) defined by �→ �→ �→ P ∈P p(x) = 4x2 + 3x 2. Then: − 4 1 1 p=Φ − 3 =Φ − (Φ (p)), sincep=4m 2 + 3m1 2m 0. B � 2� B B − − 1 0 3 Example 7.20. LetV=R 2 and choose the basis =( , ). The vectorx= V B 1 1 7 ∈ can then be represented as: � � � � � � 3 1 0 1 0 3 1 x= = 3 + 4 = =Φ − (Φ (x)) 7 1 1 1 1 4 B B � � � � � � � � � � Now, let =(c 1,...,c n) be another basis ofV. C Question: Old versus new coordinates How to switch between both coordinate vectors? α1 α1� . ? . -coordinatesΦ (v)= . -coordinatesΦ (v)= . B B ←→C C αn αn� V 1 1 Φ− Φ− B ? C Fn Fn ? 7.4 Coordinates with respect to a basis 15 n n 1 To get the map from “left to right” byf:F F ,f :=Φ Φ − . More concretely for n → C ◦ B all canonical unit vectorse j F , we get: ∈ 1 f(e j) =Φ (Φ− (ej)) =Φ (bj) (7.7) C B C Sincef is a linear map, wefind a uniquely determined matrixA such thatf(x) =Ax for allx F n. This matrix is determined by equation 7.7 and given a suitable name: ∈ Transformation matrix n n T := Φ (b1) Φ (bn) F × (7.8) C←B C ··· C ∈ The corresponding linear map gives us a sense of switching from basis to the basis . B C Also a good mnemonic is: 1 1 n Φ− T x=Φ − x for allx F (7.9) C C←B B ∈ Now, if we have a vectorv V and its coordinate vectorΦ (v) andΦ (v), respectively, ∈ B C then we can calculate: 1 T Φ (v)=Φ (Φ− (Φ (v))) =Φ (v). C←B B C B B C Wefix our result: Transformation formula Φ (v) =T Φ (v). (7.10) C C←B B v V ∈ 1 1 Φ− Φ− B T C C←B Φ (v) F n Φ (v) F n B ∈ T C ∈ B←C 1 T = (T )− . (7.11) B←C C←B 16 7 General vector spaces Rule of thumb: How to get the transformation matrixT C←B The notationT means: We put the vector in -coordinates in (from the right) C←B B and get out the vector in -coordinates. To get the transformation matrixT C C←B write the basis vectors of in -coordinates and put them as columns in a matrix. B C Example 7.21. We already know the polynomial basis =(m ,m ,m ) = (x x 2, x x, x 1) B 2 1 0 �→ �→ �→ =:b 1 =:b 2 =:b 3 in 2(R). Now, we can easily���� show���� that���� P =(m 1 m ,m + 1 m ,m ). C 2 − 2 1 2 2 1 0 =:c 1 =:c 2 =:c 3 ���� defines also a basis of 2(R). Now� �� we know� � how�� to� change between these two bases. P Therefore, we calculate the transformation matrices. Thefirst thing you should note is that the basis is already given in linear combinations of the basis vectors from . Hence C B we get: 1 1 0 1 1 = T = /2 /2 0 . B←C ⇒ −0 0 1 1 By calculating the inverse, we get the other transformation matrixT = (T )− : C←B B←C 1 /2 1 0 1 − T = Φ (b1) Φ (b2) Φ (b3) = /2 1 0 C←B C C C 0 0 1 For an arbitrary polynomialp(x) = ax 2 + bx+c with a, b, c R, we get: ∈ 1 a /2 1 0 a /2 b 1 − a − Φ (p) =T Φ (p) = /2 1 0 b = /2 +b . C C←B B 0 0 1 c c 7.4 Coordinates with respect to a basis 17 Hence, for our examplep(x) = 4x 2 + 3x 2,a=4,b=3 andc= 2, we get: − − 4 /2 3 1 4 − − Φ (p) = /2 + 3 = 5 . C 2 2 − − Let us check again if this was all correct: ( 1) (x2 1 x) +5 (x2 + 1 x) +( 2) 1 = ( 1 + 5)x2 + ( 1 + 5 )x+( 2)1 = 4x 2 + 3x 2.
Details
-
File Typepdf
-
Upload Time-
-
Content LanguagesEnglish
-
Upload UserAnonymous/Not logged-in
-
File Pages11 Page
-
File Size-