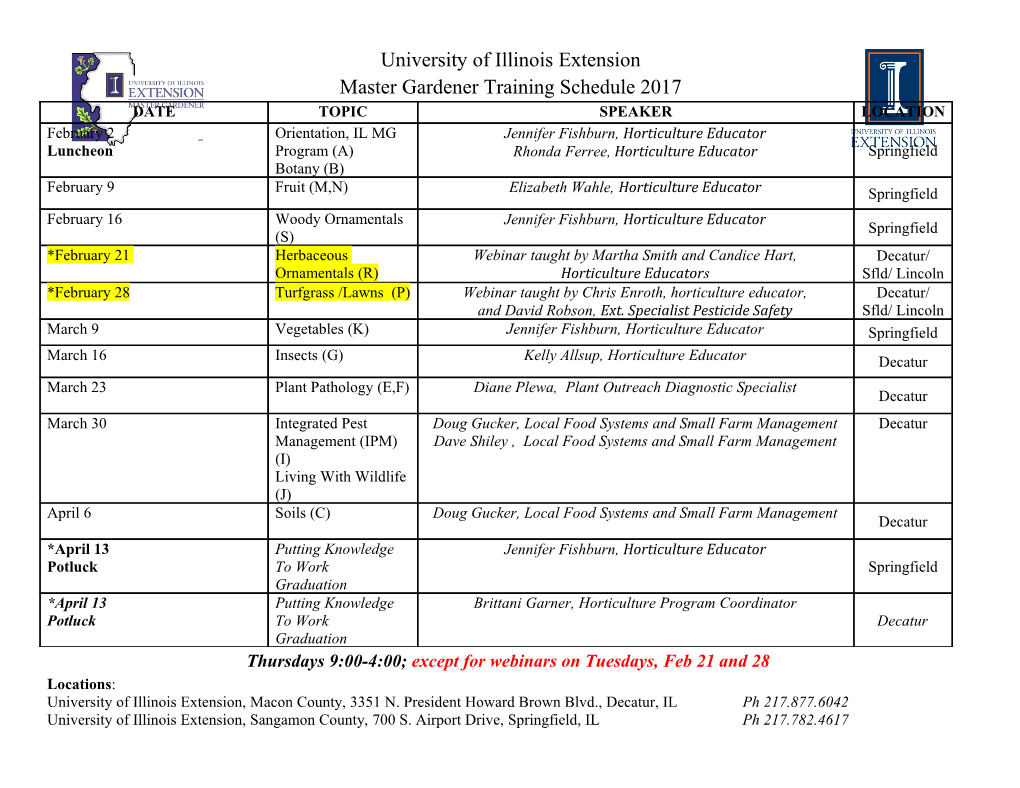
学位論文 Production and evolution of axion dark matter in the early universe 初期宇宙におけるアクシオン暗黒物質の生成 および発展について 平成24年12月 博士(理学)申請 東京大学大学院理学系研究科 物理学専攻 齋川 賢一 Abstract Axion is a hypothetical particle introduced as a solution of the strong CP problem of quan- tum chromodynamics (QCD). Various astronomical and experimental searches imply that the axion is invisible in the sense that its interactions with ordinary matters are considerably weak. Due to this weakness of the coupling, the axion is regarded as a viable candidate of dark matter of the universe. In this thesis, we investigate production and evolution of axion dark matter, and discuss their cosmological implications. Axions are produced non-thermally in the early universe. A well known production mechanism is so called the misalignment mechanism, where the axion field begins to coherently oscillate around the minimum of the potential at the time of QCD phase transition. This coherent oscillation of the axion field behaves as a cold matter in the universe. In addition to this coherent oscillation, however, there are other contributions, which come from the decay of topological defects such as strings and domain walls. The production mechanism due to topological defects is not understood quite well, and there is a theoretical uncertainty on the determination of the relic abundance of dark matter axions. In order to remove this uncertainty, we analyze the spectrum of axions radiated from these string-wall systems. The evolution of topological defects related to the axion models is investigated by performing field-theoretic lattice simulations. The spectrum of radiated axions has a peak at the low frequency, which implies that axions produced by the defects are not highly relativistic. By the use of the results of numerical simulations, the relic abundance of dark matter axions is reanalyzed including all production mechanisms. It is found that the decay of domain walls produces significant amount of cold axions, which gives severe constraints on the model parameters. In particular, for the case with short-lived domain walls, the inclusion of the domain wall contribution leads to a more severe upper bound on the axion decay constant. Furthermore, models which predict long-lived domain walls are excluded because of the overproduction of cold axions, unless an unacceptable fine-tuning exists. i Contents 1 Introduction 1 1.1 Overview . 1 1.2 Outline of this thesis . 4 1.3 Notations . 5 2 Strong CP problem and axion 6 2.1 The theta vacuum . 7 2.2 The Peccei-Quinn mechanism . 10 2.3 Phenomenological models of the axion . 12 2.3.1 The original PQWW model . 13 2.3.2 The invisible axion . 14 2.4 Properties of the invisible axion . 15 2.4.1 Mass and potential . 15 2.4.2 Coupling with other particles . 17 2.4.3 Domain wall number . 18 2.5 Search for the invisible axion . 19 2.5.1 Laboratory searches . 19 2.5.2 Astrophysical bounds . 21 2.5.3 Cosmology . 22 2.5.4 Summary – The axion window . 22 3 Axion cosmology 24 3.1 Thermal production . 24 3.2 Non-thermal production . 28 3.2.1 Evolution of the axion field . 28 3.2.2 Cold dark matter abundance . 30 3.3 Axion isocurvature fluctuations . 32 4 Axion production from topological defects 36 4.1 Formation and evolution of topological defects . 37 4.1.1 Axionic string and axionic domain wall . 37 4.1.2 Domain wall problem and its solution . 40 4.2 Evolution of string-wall networks . 41 4.2.1 Short-lived networks . 42 ii iii 4.2.2 Long-lived networks . 51 4.3 Axion production from strings . 62 4.4 Axion production from short-lived domain walls . 66 4.5 Axion production from long-lived domain walls . 75 4.5.1 Production of axions . 75 4.5.2 Production of gravitational waves . 82 4.6 Constraints for models with NDW = 1 .................... 85 4.7 Constraints for models with NDW > 1 .................... 89 4.7.1 Axion cold dark matter abundance . 89 4.7.2 Neutron electric dipole moment . 92 4.7.3 Implication for models . 93 4.7.4 Scenario with extremely small δ ................... 93 5 Conclusions and discussion 97 A Notes of standard cosmology 102 A.1 The Friedmann Equation . 102 A.2 Thermodynamics in the expanding universe . 103 A.3 Horizons . 106 B Extended field configurations 107 B.1 Classifications . 107 B.2 Instantons . 109 B.3 Symmetry restoration and phase transitions . 114 B.4 Cosmic strings . 116 B.5 Domain walls . 118 C Lattice simulation 121 C.1 Formulation . 121 C.2 Identification of topological defects . 122 C.2.1 Identification of strings . 123 C.2.2 Identification of domain walls . 123 C.2.3 Calculation of scaling parameters . 125 C.3 Calculation of power spectrum of radiated axions . 125 C.3.1 Energy spectrum of axions . 126 C.3.2 Pseudo-power spectrum estimator . 127 C.3.3 Subtraction of preexisting radiations . 129 C.4 Calculation of gravitational waves . 131 Chapter 1 Introduction 1.1 Overview In the last several decades, developments of astronomical observations have provided rich information about our universe. One of the most important accomplishments is that our universe is filled by some non-baryonic energy components. Conventionally, these are cat- egorized into two ingredients: dark matter and dark energy. Dark energy is something like the Einstein’s cosmological constant, which accelerates the expansion of the present universe. Although the accelerated expansion is observationally confirmed [1, 2], the exis- tence of constant energy density is still under debate [3]. On the other hand, the existence of dark matter is becoming more evident. The total matter density of the universe has been measured by many different kinds of methods [4], whose results are 5-6 times larger than the baryon density of the universe obtained by the observation of the light element abun- dance [5]. This indicates that the large fraction of the cosmic matter density is occupied by a non-baryonic component. This result is also confirmed by the recent precise measure- ment of cosmic microwave background (CMB) by the WMAP satellite [6]. Furthermore, the map of the gravitational potential around a cluster merger 1E0657-558, measured by means of the weak lensing, clearly shows that the matter distribution of the galaxies does not trace the distribution of the visible baryonic gas [7]. This observation strongly supports the existence of a non-baryonic matter, which interacts with ordinary matters only through the gravitational force. The existence of the dark matter cannot be explained in the framework of the standard model of particle physics. This fact motivates us to consider some new physics beyond the standard model. Several models of the particle dark matter have been proposed so far [see e.g. [8] for reviews]. One of the well-motivated candidates is the axion [9, 10]. Axion is a hypothetical particle which arises as a consequence of the Peccei-Quinn (PQ) [11, 12] mechanism, the most attractive solution to the strong CP problem of quantum chromo- dynamics (QCD) [13, 14]. This mechanism introduces a global U(1)PQ symmetry (so called PQ symmetry) that has to be spontaneously broken at some high energy scale. The spontaneous breaking of this global symmetry predicts an existence of a (pseudo) Nambu- Goldstone boson, which we identify as the axion. Historically, the axion was not considered as a candidate for the dark matter at the time 1 2 when it was proposed. In the original model, the axion was “visible” in the sense that it gives some predictions for laboratory experiments. Unfortunately, no signature was ob- served, and the prototype axion model was ruled out soon after the proposal [15]. However, it was argued that models with higher symmetry breaking scale denoted as Fa (the axion decay constant) can still avoid the experimental constraints [16, 17, 18, 19]. The essential point is that the couplings between axions and other fields are suppressed by a large fac- tor of the symmetry breaking scale ∼ 1=Fa. These models are called “invisible axions” because of their smallness of coupling with matter. This invisibleness leads to a cosmological consequence. It turns out that almost stable coherently oscillating axion fields play a role of the dark matter filled in the universe [20, 21, 22]. Furthermore, since these axions are produced non-thermally, they are cold in the sense that they are highly non-relativistic. This property agrees with the cold dark matter scenario motivated by the study of the large scale structure formation [23]. The behavior of dark mater axions is closely related with the history of the early uni- verse. In particular, the cosmological phase transition associated with the spontaneous symmetry breaking gives some implications for the physics of the axion dark matter. There are two relevant phase transitions. One is the PQ phase transition corresponding to the spontaneous breaking of U(1)PQ symmetry, and another is the QCD phase transition cor- responding to the spontaneous breaking of the chiral symmetry of quarks. Axions are produced at the PQ phase transition, then they acquire a mass due to the non-perturbative effect at the QCD phase transition. The remarkable feature of this sequence of phase tran- sitions is that it predicts the formation of topological defects [see [24] for reviews]. When the PQ symmetry is spontaneously broken, vortex-like defects, called strings, are formed. These strings are attached by surface-like defects, called domain walls, when the QCD phase transition occurs. The cosmological evolution of these topological defects is a key to understand the physics of dark mater axions. The structure of the domain walls is determined by an integer number NDW which is re- ferred as the “domain wall number”.
Details
-
File Typepdf
-
Upload Time-
-
Content LanguagesEnglish
-
Upload UserAnonymous/Not logged-in
-
File Pages152 Page
-
File Size-