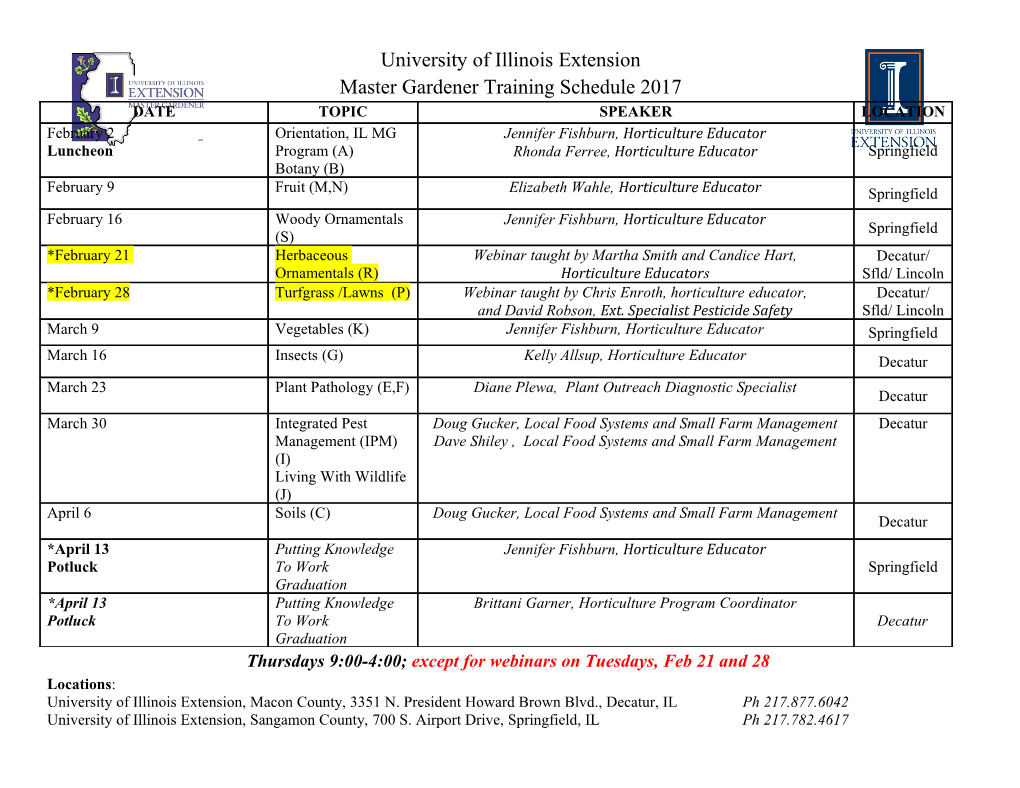
Atmos. Meas. Tech., 9, 3707–3715, 2016 www.atmos-meas-tech.net/9/3707/2016/ doi:10.5194/amt-9-3707-2016 © Author(s) 2016. CC Attribution 3.0 License. Mesospheric temperature soundings with the new, daylight-capable IAP RMR lidar Michael Gerding, Maren Kopp, Josef Höffner, Kathrin Baumgarten, and Franz-Josef Lübken Leibniz Institute of Atmospheric Physics at the Rostock University, Kühlungsborn, Germany Correspondence to: Michael Gerding ([email protected]) Received: 20 April 2016 – Published in Atmos. Meas. Tech. Discuss.: 3 May 2016 Revised: 15 July 2016 – Accepted: 19 July 2016 – Published: 11 August 2016 Abstract. Temperature measurements by lidar are an impor- 1 Introduction tant tool for the understanding of the mean state of the at- mosphere as well as the propagation of gravity waves and Temperature is one of the key parameters for the understand- thermal tides. Though, mesospheric lidar soundings are of- ing of the atmosphere. The mean state is mainly a result ten limited to nighttime conditions (e.g., solar zenith an- of the residual circulation and the radiative budget, as well gle > 96◦) due to the low signal-to-noise ratio during the as the chemical and turbulent heating and cooling. Super- day. By this, examination of long-period gravity waves and posed spatiotemporal temperature variations resulting from tides is inhibited, as well as soundings in summer at polar planetary, tidal and gravity waves are observed. Propagating latitudes. We developed a new daylight-capable Rayleigh– waves become increasingly important in the middle atmo- Mie–Raman (RMR) lidar at our site in Kühlungsborn, Ger- sphere because of decreasing air density. Observational capa- many (54◦ N, 12◦ E), that is in routine operation since 2010 bilities have improved in the last decade due to sophisticated for temperature soundings up to 90 km or ∼ 75 km (night or satellite-borne instruments like TIMED/SABER, but several day) and soundings of noctilucent clouds. Here we describe constraints remain, such as a coarse spatiotemporal resolu- the setup of the system with special emphasis on the daylight tion. While lacking global coverage, ground-based lidars are suppression methods like spatial and spectral filtering. The the only technique for observation of temporal temperature small bandwidth of the Fabry–Pérot etalons for spectral fil- variations on scales of 10–60 min in the middle atmosphere tering of the received signal induces an altitude-dependent (e.g., Meriwether and Gerrard, 2004). However, lidars suf- transmission of the detector. As a result, the signal is no fer from solar photon flux during the day, and therefore most longer proportional to the air density and the hydrostatic inte- lidar observations are limited to nighttime conditions. This gration of the profile results in systematic temperature errors hinders, e.g., measurements at polar latitudes in summer and of up to 4 K. We demonstrate a correction method and the the observation of tidal temperature variations, and it may validity of correction by comparison with data obtained by also bias the retrieval of the seasonal temperature variation our co-located, nighttime-only RMR lidar where no etalon is due to unresolved tides (Chen et al., 2000). However, a po- installed. As a further example a time series of temperature tential bias might affect all data sets with limited temporal profiles between 20 and 80 km is presented for day and night coverage such as sun-synchronous orbiting satellites. of 9–10 March 2014. Together with the other data of March A few daylight-capable systems have been developed spe- 2014 these profiles are used to calculate tidal amplitudes. It cially for resonance lidars, often using atomic vapor cells is found that tidal amplitudes vary between ∼ 1 and 5 K de- (e.g., Chen et al., 1996; Fricke-Begemann et al., 2002; Fried- pending on altitude. man et al., 2012) or Fabry–Pérot etalons (FPEs; e.g., Chu et al., 2002) for spectral filtering of the received signal. These lidars are typically limited to the 85–105 km altitude range. Below, Rayleigh backscatter is used for temperature retrieval. Several systems cover the whole range up to ∼ 85 km at night, but for daylight operation the range is typically lim- Published by Copernicus Publications on behalf of the European Geosciences Union. 3708 M. Gerding et al.: Daylight-capable RMR lidar ited to ∼ 60 km (e.g., von Zahn et al., 2000; Klekociuk et al., 2003; Blum and Fricke, 2005) if available at all. While all SM2 these daylight-capable lidars are installed at polar latitudes, TO Detector there is a general lack of temperature data during day from SM1 mid-latitudes, especially for the mesospheric altitudes above 60 km. BWT We developed a daylight-capable Rayleigh–Mie–Raman BD BD: beam dump (RMR) lidar at Leibniz-Institute of Atmospheric Physics BWT:beam expander ◦ ◦ Quanta-Ray PRO290–30 Pwr: powermeter (IAP) in Kühlungsborn, Germany (54 N, 12 E), in (1064 nm, 532 nm) Pwr Shutter SM: steering mirror 2009/2010. The new system has already proven to observe TO: telescope optics noctilucent clouds (NLCs; also known as polar mesospheric l-Meter clouds) during day and night (Gerding et al., 2013b) as well Prometheus 50NE as tidal temperature variations (Kopp et al., 2015). Sound- (1064 nm, 532 nm) ◦ I Cell ings have been performed at solar elevations of up to 59 , 2 i.e., the maximum elevation during summer. The new lidar Figure 1. Setup of the transmitter of the new RMR lidar. Only the is co-located with a nighttime-only RMR lidar that has been most important fiber cables of the seed laser are shown; additional in operation since 1997, e.g., for observation of temperatures fibers are available, e.g., for adjustment of the detector. SM1 is (Alpers et al., 2004; Gerding et al., 2008; Rauthe et al., 2008) Piezo-mounted for fast beam stabilization, and SM2 is motorized and NLCs (Alpers et al., 2000; Gerding et al., 2013a). for coarse adjustment of the beam axis. Here we describe the instrumental setup of the new RMR lidar at Kühlungsborn (Sect. 2). We focus on the special re- quirements for daytime operation. Narrowband spectral fil- – widely automated system (e.g., active beam stabiliza- tering entails a somewhat different temperature retrieval, as tion), allowing regular observations even with briefly the backscatter signal is no longer proportional to the air den- trained staff; sity (see Sect. 3). We describe the temperature retrieval for – operation at broken clouds. the new lidar and compare the results exemplarily with data simultaneously obtained with the old RMR lidar. In Sect. 4 The new lidar is optimized for soundings of middle atmo- we present an example for temperature lidar soundings cov- spheric temperatures and NLCs during both day and night. ering day and night. We also show tidal temperature vari- Details of the instrumental setup are shown in Figs.1 and2. ations for this period, affirming the results of Kopp et al. The flashlamp-pumped power laser Newport Spectra (2015). In some examples the data of the RMR lidar are com- Physics Quanta-Ray PRO 290-30 is seeded by the continuous plemented by observations with the co-located potassium wave laser Innolight Prometheus 50NE (Fig.1). The external resonance lidar (von Zahn and Höffner, 1996). The potas- seed laser allows a better wavelength control and wavelength sium lidar allows daytime soundings since year 2000 (e.g., monitoring compared to an internal seed laser. For this, it is Fricke-Begemann and Höffner, 2005). locked to an iodine line by means of absorption spectroscopy using the frequency-doubled output of the seed laser. Un- til June 2015 the iodine line 1111 (λvac D 532:241 nm) was 2 Instrumental setup of the new IAP RMR lidar used. Since then we use line 1109 at λvac D 532:255 nm, cor- responding to a seeding wavelength λ D 1064:51 nm We installed a new RMR lidar at Kühlungsborn in 2009/2010 seed,vac or λ D 1064:22 nm for vacuum and air respectively. and started regular operation in summer 2010. The design seed,air The new seeding wavelength results in the advantage of a requirements for the RMR lidar are as follows: second harmonic “in air” (λair D 532:11 nm) being identical – reduction of background count rate by more than 4 or- with a Fraunhofer line in the solar spectrum, i.e., a ∼ 25 % ders of magnitude with a combination of spatial filtering reduced solar background. The emission wavelength of the (narrow field of view) and spectral filtering (narrowband seed laser is monitored by a High Finesse WSU wavelength detector); meter, while the fine control of the emission wavelength is done by means of the above mentioned iodine absorption – co-axial transmitter and receiver for complete overlap in spectroscopy. Additional fiber ports of the seeding system the stratosphere and mesosphere; are used for adjustment of the optical bench, for seeder power – high dynamic range of the detector system for signal monitoring, etc. Due to the low signal-to-noise ratio (SNR) coverage between ∼ 20 and 90 km; we actually only use the second harmonic at 532 nm and not the fundamental laser output at 1064 nm. The frequency- – detection of elastic (532 nm) and inelastic (N2-Raman doubled output of the power laser is guided through a 10× shifted, 608 nm) backscatter for future aerosol correc- beam widening telescope (BWT) for reduction of the beam tion in the lower stratosphere; divergence from < 0.5 to 0.05 mrad. Two steering mirrors Atmos. Meas. Tech., 9, 3707–3715, 2016 www.atmos-meas-tech.net/9/3707/2016/ M. Gerding et al.: Daylight-capable RMR lidar 3709 608 APD APD PMT 532/2 532/1 IF532 IF608 Chopper APD 532/3 IF532 5 % R 5 % R IF532 HR608/ Fiber cable FPE 2 FPE 1 HT532 from telescope Figure 2.
Details
-
File Typepdf
-
Upload Time-
-
Content LanguagesEnglish
-
Upload UserAnonymous/Not logged-in
-
File Pages9 Page
-
File Size-