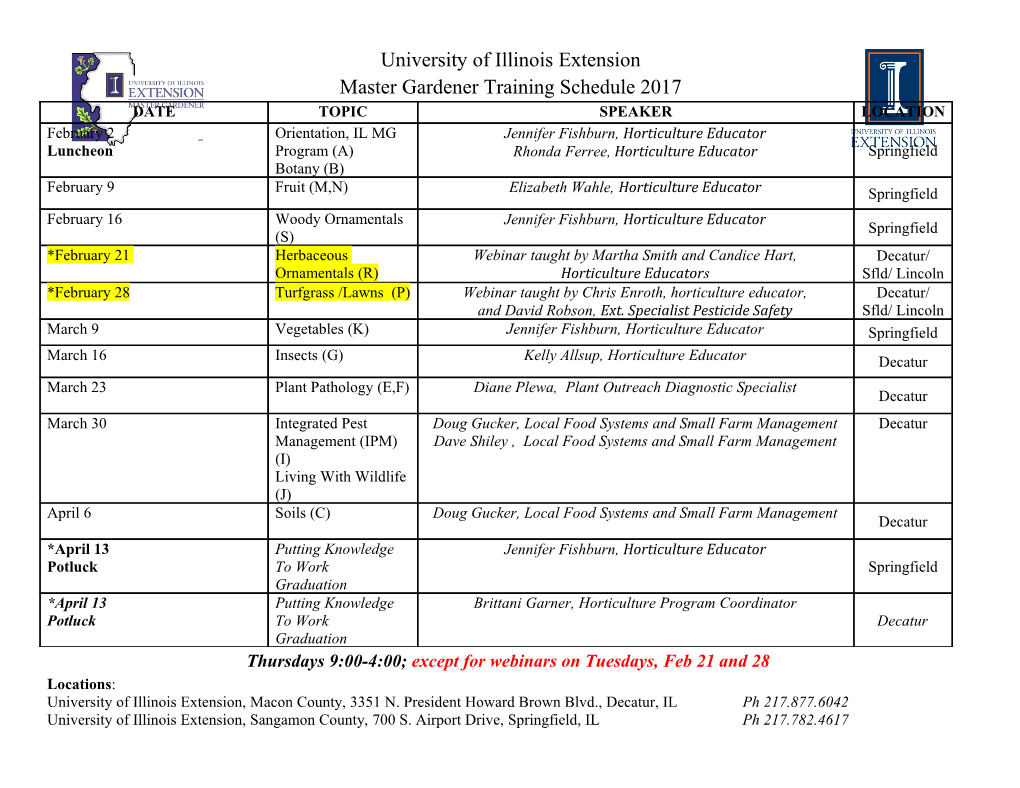
Keynote presentation The roots and tops of magnetite-apatite mineralization: evolving magmatic-hydrothermal systems Fernando Tornos IGEO, Consejo Superior de Investigaciones Científicas, Spain John M. Hanchar Memorial University of Newfoundland, St-John's, Canada Francisco Velasco Universidad del País Vasco UPV/EHU, Spain Rodrigo Muñizaga Compañía Minera del Pacífico, Chile Gilles Levresse Universidad Autónoma de México, México Abstract. Magnetite-apatite deposits form a well-defined Cordillera of the Andes, Missouri, the Urals, China, and type of mineralization characterized by the presence of a Iran. Furthermore, some of them are potential sources of core of massive magnetite ± apatite with an extensive Co and REE. Despite grouped within the Iron Oxide- aureole of alkali-calcic alteration hosting a low grade Copper-Gold (IOCG) style of mineralization (Williams et mineralization. Geology and geochemistry suggest that al. 2005), MtAp deposits form an independent group that these deposits are the product of the crystallization of oxidized water-bearing iron-rich melts that separated from a is easily discernible from the dominantly replacive sensu parental silicate magma. The temporal and vertical stricto IOCG deposits. evolution of these systems is controlled by the timing and The existence of melt inclusions and crystallized depth of separation of large amounts of aqueous fluids, a magmas (nelsonite) with a composition similar to MtAp process that leads to the formation of complex magmatic- deposits, the high temperature of formation, the geologic hydrothermal systems. These magnetite-apatite systems relationships and the common presence of structures grade upwards into bodies of massive apatite or an similar to that of crystallized igneous rocks suggest that extrusive magnetite-apatite mineralization. A key feature of these rocks are the product of the crystallization of iron- this style of mineralization is the formation of large breccia rich melts (Frietsch 1978; Naslund et al. 2002; Tornos et pipes and diatremes that are related to melt degassing at shallow depths. al. 2016). 1 Magnetite-apatite deposits 2 The roots of the system: Immiscible iron- rich melts Magnetite-apatite (MtAp) rocks form a well-defined group of ore deposits characterized by the presence of massive There is widespread evidence that immiscible P-bearing magnetite with variable amounts of F-apatite, iron-rich melts are present in different magmatic systems diopside/actinolite, and scapolite; this type of massive within the Earth’s crust (e.g., Clark and Kontak 2004; orebody has sharp non-replacive contacts with the host Jakobsen et al. 2005). Recent work (Kamenetsky et al. rocks, which are usually affected by a large aureole of 2013) shows that there is a solvus surface between Fe-rich alkali-calcic hydrothermal alteration that is zoned around melts enriched in Ca, Mg, Ti and P, and silica-rich melts the massive mineralization. They typically have a enriched in Al, Na and K. Water and volatiles are strongly characteristic mineral assemblage including K fractionated into the Fe-rich end member (Lester et al. feldspar/albite, diopside/actinolite and scapolite with a 2013). The ultimate cause of melt immiscibility can be low grade-mineralization in breccias, stockworks or crustal contamination by P-, Fe- or silica-rich rocks disseminated ore. All these deposits are poor in sulphides (Philpotts 1967). In the Andes, the Sr-Nd isotope and quartz but have variable amounts of anhydrite. In geochemistry of MtAp ores tracks the composition of the detail, the massive magnetite mineralization form vertical underlying basement and in several sites it is likely that the ellipsoidal pipes or lenses controlled by major transcrustal primitive andesitic magmatism interacted with P-rich faults; only when located at or near the surface form evaporite-bearing sedimentary rocks. stratiform deposits. The shape of the solvus is critical for the formation of Despite not being as economically significant as the MtAp mineralization; only systems crystallizing at banded iron formations (BIF), they can form large decreasing temperatures or with high degrees of crustal deposits such as Kiruna (Sweden) and as well as several contamination will form immiscible iron-rich and rhyolite other medium-sized ones clustered in the Coastal SY03 – IOCG-IOA ore systems and their magmatic-hydrothermal continuum: A family reunion? 831 melts. Otherwise, the immiscible melts will have Magnetite occurring as microphenocrysts or in melt intermediate compositions; dacite-andesite and pyroxene- inclusions in the parental rocks, as well as sometimes in magnetite. This seems to be a critical feature of these the alkali-calcic hydrothermal assemblage, are enriched in systems, since only iron-rich melts having low Si contents Ti (≈>2 wt%), commonly containing exsolution lamelae of – and hence low viscosities but high density – can Ti-rich oxides (Velasco et al. 2016). These major effectively separate from the much more viscous silicate differences cannot be due solely to changes in the melt that should be left behind. Otherwise, it is likely that temperature-fO2 but are interpreted as controlled by the KD the two immiscible components do not separate. Evidence between the iron-rich melt and the exsolving volatile (P- or of the existence of a silica-rich melt that should accompany F-rich) hydrothermal fluids or by mineral equilibria the magnetite-apatite mineralization are rare. However, involving Ti-bearing magnetite, titanite or rutile. The last some have been found near several MtAp deposits like Los two minerals are widespread in the massive MtAp Colorados, La Perla, El Laco in Chile and Rektorn mineralization and the host rock hydrothermal alteration. (Kiruna, Sweden). In the Coastal Cordillera of the Andes, the MtAp deposits are located along the major Atacama Fault System, which behaves as a strike-slip structure during the ore forming event in the Late Jurassic-Early Cretaceous (Sillitoe 2003). As in many other magmatic-hydrothermal systems, the formation of small zones of vertical extension such as pull-apart structures or fault splays should promote the upwards intrusion of melts and fluids to shallow depths. Density-controlled buoyancy seems to play a minor role in this part of the system. Locally, near these deposits there are dykes of microdiorite with up to cm-sized drops of magnetite that are here interpreted as crystallized remnants of the iron- rich melt trapped prior to coalescence. They show no appreciable chemical zoning but complex intergrowths with titanite (Fig. 1). The water-rich nature of these melts is evidenced by the presence of an aureole of hydrothermal Figure 1. Drops of magnetite showing no chemical zonation and alteration. enclosed in an aureole of hydrothermal (alkali-calcic) alteration w the host microdiorite. Magnetite shows abundant exsolution lamelae of ülvospinel and is intergrown with titanite (Los 3 Water separation and melt crystallization Colorados Mine, Chile). The solidus temperature of an iron-rich melt strongly If iron-rich melts behave similar to silicate melts, the depends on the phosphorous, fluorine, and water contents, ascent and later crystallization of the magnetite rock which significantly lower the temperature of should promote the exsolution of large amounts of aqueous crystallization from ca. 1600°C to less than 1000°C. The fluids enriched in incompatible elements (Na, K), volatiles crystallization of an almost monomineralic magnetite rock and abundant Fe. As in other magmatic-hydrothermal is due to the high oxygen fugacity and low aSiO2 of the systems, when water exsolution takes place at intermediate melt, something that inhibits the formation of most iron- to shallow depths (i.e., <4 km) and above the two-phase bearing silicates; when present, they often form an surface, it will separate in two immiscible phases, a external zone of coarse-grained actinolite accompanied by dominant low density vapour carrying most of the volatiles interstitial magnetite. After the crystallization of and a residual relatively immobile brine enriched in magnetite, only apatite and small amounts of elements that form chloride complexes (Driesner and actinolite/diopside can crystallize. Heinrich 2007); the relative proportion, and the salinity, of One of the most striking features of these deposits is the immiscible fluids depends on the depth but in very the highly variable Ti content of the magnetite. While the shallow environments this process can form small amounts classical nelsonite has Ti-rich magnetite, magnetite of the of an (hydro)-saline melt as has been described in El Laco MtAp mineralization is usually regarded as impoverished by Broman et al (1999) accompanied by large amounts in Ti (≈<5000 µg/g) (Dare et al. 2015; Knipping et al. (>99 wt%) of a low density gas. Reaction of the high 2015; Velasco et al. 2016; Broughm et al. 2017; density chloride-rich brine with the host rock would Kołodziejczyk et al. 2017). Despite that is generally true, produce the alkali-calcic alteration observed around the the Ti content of magnetite crystallized from immiscible massive magnetite-apatite zone and associated low grade melts can be very low as has been described in several mineralization while low-density gas would tend to flow localities. In MtAp deposits it varies significantly between upward. A mixture of gas with the melt would ascend in a the different generations of magnetite crystallization. form similar to that described by Woods and Cardoso 832 Mineral
Details
-
File Typepdf
-
Upload Time-
-
Content LanguagesEnglish
-
Upload UserAnonymous/Not logged-in
-
File Pages4 Page
-
File Size-