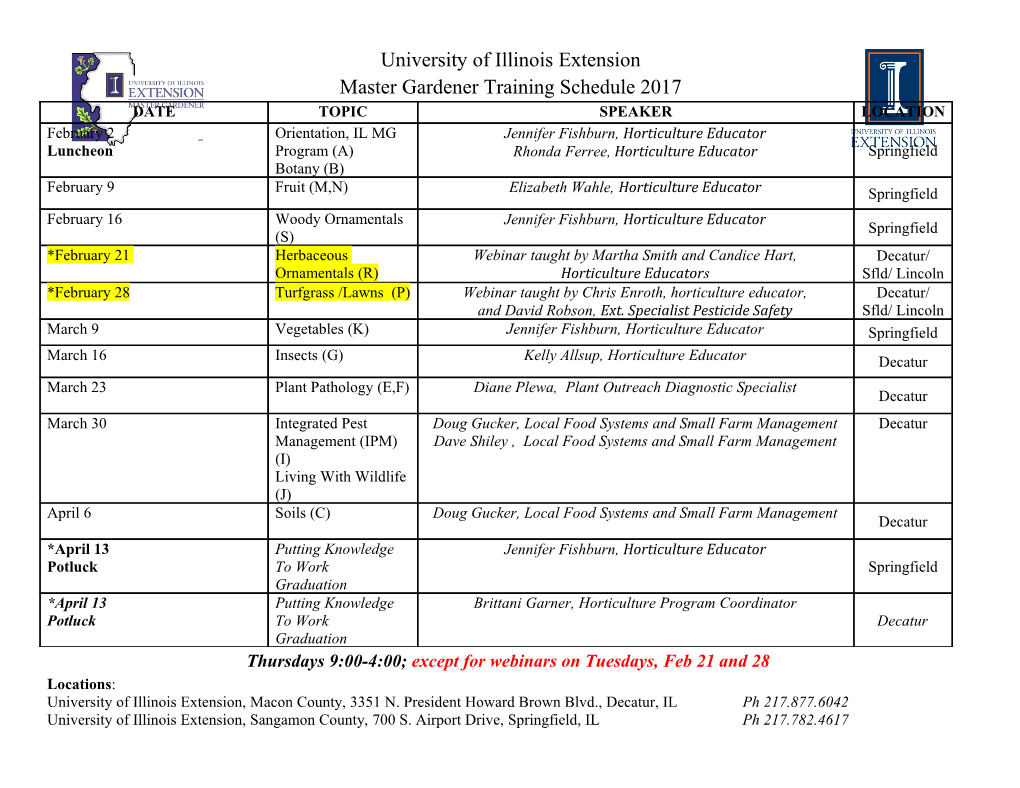
Solid-State Electronics Vol. 35, No. 3, pp. 325-332, 1992 0038-1101/92 $5.00+ 0.00 Printed in Great Britain. All rights reserved Copyright © 1992 Pergamon Press pie ULTRAHIGH-BANDWIDTH VECTOR NETWORK ANALYZER BASED ON EXTERNAL ELECTRO-OPTIC SAMPLING M. Y. FRANKEL, J. F. WHITAKER, G. A. MOUROU and J. A. VALDMANIS Ultrafast Science Laboratory, Department of Electrical Engineering and Computer Science, University of Michigan, Ann Arbor, MI 48109-2099, U.S.A. (Received 31 July 1991) Abstract--We report the development of an ultrahigh-bandwidth vector network analyzer useful for small-signal characterization of high-speed semiconductor devices. It employs 100-fs optical pulses for making terahertz-bandwidth electro-optic measurements of electrical signals, as well as for sub-picosec- ond, photoconductive, electrical-stimulus-signalgeneration. High-bandwidth coplanar strip transmission lines are used for signal transmission. A 0.15 x 50gm gate A1GaAs/InGaAs/GaAs HFET has been characterized over a bandwidth of 100 GHz using this network analyzer. A comparison with conventional RF network analyzer measurements performed to 40 GHz demonstrated good agreement throughout this bandwidth. Such measurements of the actual device characteristics across their entire operating frequency range should improve device development and incorporation into active circuits. NOTATION scopes are not effective at such high frequencies, the major limitation being imposed by the connectors ~t(f) frequency-dependent attenuation factor fl(f) frequency-dependent phase factor and waveguides needed for signal coupling between d substrate thickness the instrument and the device under test (DUT). This Esos sapphire relative permittivity lack of convenient and accurate high-bandwidth Esup effectivesuperstrate relative permittivity including device characterization methods imposes a serious the effect of an external electro-optic probe f~x transistor maximum frequency of oscillation obstacle to progress in semiconductor device S coplanar strip transmission line spacing between development and utilization. conductors The recent development of ultrafast optoelectronic tr coplanar strip transmission line conductor d.c. con- techniques has allowed novel high-bandwidth device ductivity characterization to be implemented[2-7]. These St1 forward reflection scattering parameter St2 reverse transmission scattering parameter techniques rely on the unrivaled high-speed electrical $21 forward transmission scattering parameter signal generation and measurement capabilities t coplanar strip transmission line conductor thickness provided by ultrashort optical pulses--50fs-10.ps V~ actual incident electrical signal duration--emitted by various lasers. The photocon- V~ measured incident electrical signal V~r measured reflected electrical signal ductive switching method[8] is used to generate pi- V~,t measured transmitted electrical signal cosecond-duration electrical stimulus signals which V r actual reflected electrical signal have bandwidths that extend for hundreds of giga- Vt actual transmitted electrical signal hertz. For electrical measurements over similar band- W coplanar strip transmission line conductor width widths, either photoconductive[8] or electro-optic[9] z electricalsignal propagation distance ZEEp perturbed transmission line characteristic impedance sampling may be used, where the ultimate response of Z0 unperturbed transmission line characteristic such techniques is limited by the duration of the impedance photoconductively generated electrical stimulus sig- nal, the bandwidth of the interconnecting trans- 1. INTRODUCTION mission lines carrying the signals to be measured and the duration of the optoelectronic sampling gate. The continuing advances in the development of mod- There are two significant advantages that opto- ern semiconductor devices have pushed the response electronic techniques possess over conventional, of such devices to frequencies in excess of 400 GHz[1]. purely electronic ones. First, optoelectronic electrical Frequency-domain characterization across this entire signal generation and measurement bandwidths far bandwidth range would be one requirement for exceed those achievable electronically. Secondly, both facilitating further improvements and applications the stimulus-generation site and response-measure- for devices. However, conventional, purely electronic ment planes are physically close to the DUT, so measurement instruments such as vector network that it is possible to completely eliminate any inter- analyzers, spectrum analyzers and sampling oseillo- connection discontinuities (transitions), as well as the ss~ 35/~-~ 325 326 M.Y. FRANKELet al. errors and uncertainties introduced by the device CPM laser is currently the best source of ultrashort de-embedding procedures. Optoelectronic techniques pulses in the visible region of the spectrum, this laser have been applied and demonstrated up to 100 GHz, has been used in this investigation. The first beam is and the measurement instruments based on these acousto-optically modulated at 3.5 MHz and used to techniques should provide a viable complement to periodically excite a photoconductive switch. The electronic ones. second beam goes through the external electro-optic In this paper we describe the application of the probe (Terametrics Model 200-TIR transducer) to external electro-optic sampling (EEOS) technique[9] gate the electric-field-induced birefringence which for the extraction of small-signal S-parameters of an creates a polarization change in the probe[9]. active device. When combined with a high-band- The beam polarization change is converted via a width, coplanar-strip-transmission-line (CPS) test Wollaston-prism analyzer to an intensity change fixture[10], this technique allows one to determine detectable by the photodiodes. A compensator intro- device small-signal, frequency-domain characteristics duces additional birefringence to bias the intensity over a range of 100 GHz, although it may also be transmission function of this modulator to its 50% extended to significantly higher frequencies. In point, thereby making the intensity change linearly Section 2, we discuss the parameters and the results proportional to the local microwave electric field affecting the selection of electrical signal generation, strength in the probe. The electro-optic probe and measurement and propagation methods. Based on probing beam can be positioned to interrogate electri- these results, we have developed a complete small- cal signals appearing anywhere in the test fixture. A signal device characterization procedure. In slowly varying time-delay is introduced between the Section 3, we demonstrate the implementation of this excitation and the probing laser pulses by passing the procedure to obtain device time-domain and fre- probing beam through an optical delay rail; hence, a quency-domain characteristics. time-domain sampling scheme is realized. A low-noise audio-frequency lock-in is used in 2. OPTOELECTRONIC SYSTEM conjunction with a front-end RF mixer to reduce the signal-to-noise threshold of the described system to Figure l shows the overall measurement system sub-millivolt levels[12]. schematic. The optical pulse source is a balanced The photoconductor switch, used to generate elec- colliding-pulse mode-locked (CPM) laser which pro- trical stimulus transients[8], consists of a gap within vides two 100-fs pulse-trains at a 100 MHz repetition one of the metal transmission-line electrodes de- rate and a wavelength of 620 nm[l 1]. Since the optical posited on a semiconductor substrate. The switch gap pulse duration places a fundamental limit on the typically has a very high dark resistance and is d.c. achievable measurement-system bandwidth, and the biased. The laser excitation pulse, with photon energy Outputpulse CPM Laser J Excitatior ~ beam I beam Delay Beam {~ ~--~ rail chopper ~'"~I ~ Polarizer I I Sy,mulus Photoconductor I I signal switch Jr ~ "~ [ Device under test (DUT) dcu switch i "T"~ Electro-optic I bias _ . ~nsducer Compensatorc~_ X-axis Analyzer [~P-m~,,~ Lockin I Photoaioaes ~ ~ averager Fig. I. Optoelectronic device characterization system schematic. Ultrahigh-bandwidth vector network analyzer 327 above that of the bandgap of the photoconductor, arrangement have been investigated[15], and it has illuminates the gap, is absorbed, and creates an been shown that a properly designed external probe electron-hole plasma, thereby electrically shorting allows accurate measurements to be made with the gap. Thus, an electrical transient signal which terahertz bandwidth. propagates along the transmission line is generated. Several perturbations in a propagating electrical Three parameters affect the electrical bandwidth of waveform can result from the interaction of the this transient signal--electron-hole pair generation electrical signal with the probe tip. First, reflections rate, electron-hole pair lifetime and switch RC time at the points where the guided waveform enter and constant. The generation of the electron-hole pairs in exit the EEP input and output discontinuities are the semiconductor follows the incident optical pulse linear and cancel out in the frequency domain, where envelope to within the limitations imposed by the only the relative difference in the signals detected at uncertainty principle, assuming the optical pulse two different measurement points is typically desired. spectrum to be within the semiconductor absorption However, perturbations have to be accounted
Details
-
File Typepdf
-
Upload Time-
-
Content LanguagesEnglish
-
Upload UserAnonymous/Not logged-in
-
File Pages8 Page
-
File Size-