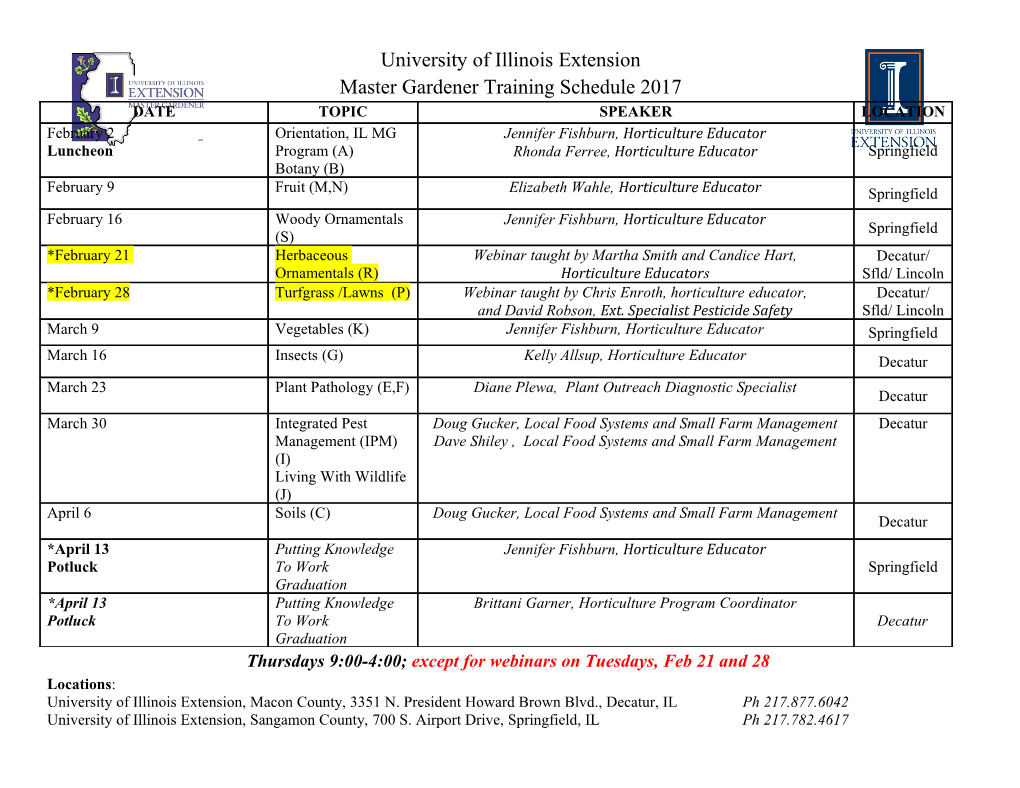
POLAROGRAPHY OF BIOLOGICAL PURINES AND PYRIMIDINES Philip 3. Elving The University of Michigan Ann Arbor, Mich. INTRODUCTION Since electron-transfer reactions are so important in biological systems, information which can be obtained in respect to the energy levels (electrical potentials) and reaction pathways involved should be helpful in the under- standing, control and utilization of such reactions. Polarographically deter- minded half-wave potentials ( and electrode reaction paths or mechanisms readily provide such data under conditions generally similar to those en- countered in biological systems, e.g., electrolytic reductions and oxidations often occur under conditions resembling those of enzymatic transformations: electron-transfer at a heterogeneous interface, previous occurrence of adsorp- tion and/or adduct formation, dilute aqueous solution, pH between 2 and 9, and moderate temperature. Postulation of the extent of correlation that may exist between electrolytic and biological redox processes must at present be largely speculation. Never- theless, to the extent that the two kinds of processes occur under similar conditions, information obtained from the examination by electrochemical techniques of the redox behavior of compounds of biological interest may help to clarify and explain biological processes with respect to the nature of the factors, such as pH, controlling the electron-transfer process. The value of electrical measurement in elucidating redox behavior of biologically important organic compounds has long been recognized. However, due to the fact that few organic redox systems behave reversibly, valid potentiometric data have been obtained for only a limited number of com- pounds. Where such data have been systematically gathered and analyzed, the results have been fruitful, e.g., the work of Michaelis, Clark and their couaborators during the 1920's and 1930's. Since then, most studies of the redox behavior of organic compounds have been based on polarography, generally at the dropping mercury electrode (D.M.E.) . Frequently, Elj2data on organic compounds so determined are the only energetic data readily obtainable by electrochemical measurement. The present paper summarizes the results of polarographic examination of purines and pyrimidines in aqueous solution with emphasis on (a) the reaction path involved in electrochemical reduction and oxidation, (b) the effect of substituents and structure in altering the ease of reduction and the reaction path, and (c) the correlation of E,,? data with electronic and other indices. The stress on reduction is simply due to the fact that, until relatively recently, systematic study of the electrochemical behavior of organic compounds was largely restricted to their reduction at mercury electrodes due to the lack of an electrode suitable for studying oxidation. In addition to classical direct-current constant-potential polarography at the D.M.E., derived techniques-controlled electrode potential electrolysis and coulometry, cyclic voltammetry, chronopotentiometry and alternating current polarography at mercury and graphite electrodes-have been used to obtain the data to be discussed. The polarography of purines and pyrimidines was reviewed several years 124 Elving: Polarography of Purines and Pyrimidines 125 ago'; the present discussion largely assumes the availability of this review. Data for the polarographic reduction of selected pyrimidines and purines and for the oxidation of purines are summarized in TABLES1 and 2. GENERALREACTION PATH Elving and Pullman2 proposed a general mechanistic pattern for organic electrode processes, based on the fact that the fundamental process in an organic electrochemical reduction is bond rupture, which requires only one electron to produce a free radical species; addition of a second electron completes rupture of the bond to give a carbanion. The pattern (FIGURE1) assumes a generalized reaction site, R:X, where R represents the reactive carbon center in the molecule and X represents another carbon, oxygen, nitro- gen, halogen or other atom; there may be more than one bond between R and X, for example, in a C=N linkage. In the primary step of the electrode process, the reaction site accepts a single electron to form the electrode-activated complex, which can either revert to the original species or dissociate to give a free radical precursor and an anionic species; the latter, if a multiple bond was originally present between R and X, could form a single, free radical anion. The exact nature of these species will be modified by the extent of the participation of protons, solvent molecules and other solution constituents or even the electrode surface (such participation in subsequent steps is not explicitly indicated but may be involved). The free radical precursor can either accept a second electron immediately on formation and be reduced to the equivalent of a carbanion, or can exist as a stable, free radical species, which may then either dimerize or, at more negative potential, be further reduced to a carbanion. The charge on the carbanion, formed by either path, is neutralized either by acceptance of a R:X + e 2 (%O, H+, etc.) = electrode complex - I Dimer EL ec tronic rearrangement products FIGURE1. Generalized mechanistic path for an organic electrode reaction, in which R:X represents the reaction site; the individual steps are discussed in the text. Allowance may have to be made for (a) the participation in the various steps of protons, solvent, other solution constituents and the electrode surface, (b) the occur- rence of chemical reactions preceding, accompanying and following charge-transfer, and (c) resulting modification of the species shown. 126 Annals New York Academy of Sciences TABLE1 POLARCGRAPHIC REDUCTIONOF SELECTED PYRIMIDINES AND PURINES AT THE DROPPINGMERCURY ELECTRODE” Compound PH El,?h IC V. Pyrimidine 0.5-5 I -0.5764.105 pH 2.G2.5 3-5 11 -1.142-0.011 pH 2.2-2.7 5-8 111 -0.680-0.089 pH 4.3-4.7 7-8 IV -1.oo(M.~5 pH 4.8 9-1 3 V -0.8054.079 pH 8.9d 2-Amino- 2-3 1 -0.6854.049 pH 2.0 4-7 I -0.425-0.121 pH 2.1-2.4 4-7 I1 -1.360-0.004 pH 2.C2.4 7-9 111 -0.680-0.090 pH 4.G4.3 2-Amino-4-met hyl- 2-4 I -0.770-0.063 pH 2.0 4-7 1-0.550-0.113 pH 2.1 5-7 11 -1.424-0.008 pH 2.0 7-9 111 -0.745-0.094 pH 4.0 2-Hydroxy- 2-8 -0.530-0.078 pH 2.1 4-Amino-2-hydroxy- (cytosine) 5.5 4.9 4-6 -1.125-0.075 pH 5 6.4 4-Amino-6-hydroxy- 1.2 -1.18 6.8 -1.62 4-Amino-2, 6-dimethyl 2-8 -1.130-0.073 pH 2- 5 6.8 4-Amino-2, 5-dimethyl 3-6 -1.11-0.076 pH 4.8 4-H ydroxy- 1.2 -1.16 6.8 -1.60 ~~ 2, 4-Diamino- 1.2 -1.16 6.8 -1.52 4, 2 6-Triamino- 1.2 -1.17 6.8 -1.57 1, 4-0-Dimethyl- (thymine) 1.2 -1.18 6.8 -1.62 Purine 2-6 I -0.697-0.083 pH 3.8 2-6 11 -0.902-0.080 pH 6.3 6-Amino- (adenine) 1-3 e 10.2 4-6 10.2 2-6 -0.9754.090 DH 5.6 6-Hydroxy- (hypoxanthine) 5.7 -1.61 2.75 Data selected from TABLES2 and 3 of Reference 1, where references to the original sources are given. Roman numbers indicate successive polarographic waves; potentials are vs. S.C.E. c Diffusion current constant, I = il/Cm2’3r1’6; each electron transferred per molecule reduced or oxidized contributes a value of about 2. The values for purine and adenine are slightly high due to the presence of some concomitant hydrogen ion reduction (cf. References 4 and 22). At pH 13, I = 6. c Dependence of El,r on pH is similar to that given for pH range of 2-6, which is for Mcllvaine buffers; these two sets of data are for chloride and acetate buffers, respectively. Elving: Polarography of Purines and Pyrimidines 127 TABLE2 VOLTAMMETRICOXIDATION OF SELECTEDPURINES AT THE STATIONARYGRAPHITE ELECTRODE^ Ratiob ED/ 1 i,/C Compound PH V. NmM Purine (no wave) 6-Amino- (adenine) 2.3-5.7 73= 5.5 1.03 to 1.07 48d 2-Amino-6-hydroxy- (guanine) 2M HzS04 1.02 50 BAmino-2-hydroxv- (isoauanine) 2M HSOa 1.05 58 6-Hydroxy- (hypoxanthine) 2.3-5.7 1.25 to 1.04 62 2, 6-Dihydroxy-(xanthine) 2M HzS04 1.01 49 3.7-5.7 0.84 to 0.71 2, 6, 8-Trihydroxy- (uric acid) 2M HzS01 0.62 3.7 0.45 31 5.7 0.33 34 a Data taken from Reference 22, with the exceptions noted in footnotes c and d. Current values are those obtained at a 0.25-inch diameter, wax-impregnated graphite rod. In the pH range of 2-5, ED at a pyrolytic graphite electrode = 1.45-0.067 pH, with i,/C being 68 for a 0.25-inch electrode (Reference 28). Obtained with a highly damped polarograph. (Reference 38). proton from the solution or by electronic rearrangement that transfers the charge to another part of the molecule where it can be suitably handled. Chemical reactions preceding, accompanying or following charge-transfer may-and often do-play significant roles in the overall process, as is sub- sequently indicated. Reduction of the Pyritnidine Ring The complex pattern observed for the reduction of pyrimidine at the D.M.E., in which five polarographic waves appear over the normal pH range (cf. TABLEl), can be explained3 on the basis of the general electrode reaction mechanism described (FIGURE2): Wave I results from le reduction of the 3, 4 bond with the simultaneous acquisition of a proton to form a free radical, which may dimerize to 4,4'-bipyrimidine or be reduced at more negative potential in a further le process (wave 11) to 3, 4- dihydropyrimidine.
Details
-
File Typepdf
-
Upload Time-
-
Content LanguagesEnglish
-
Upload UserAnonymous/Not logged-in
-
File Pages16 Page
-
File Size-