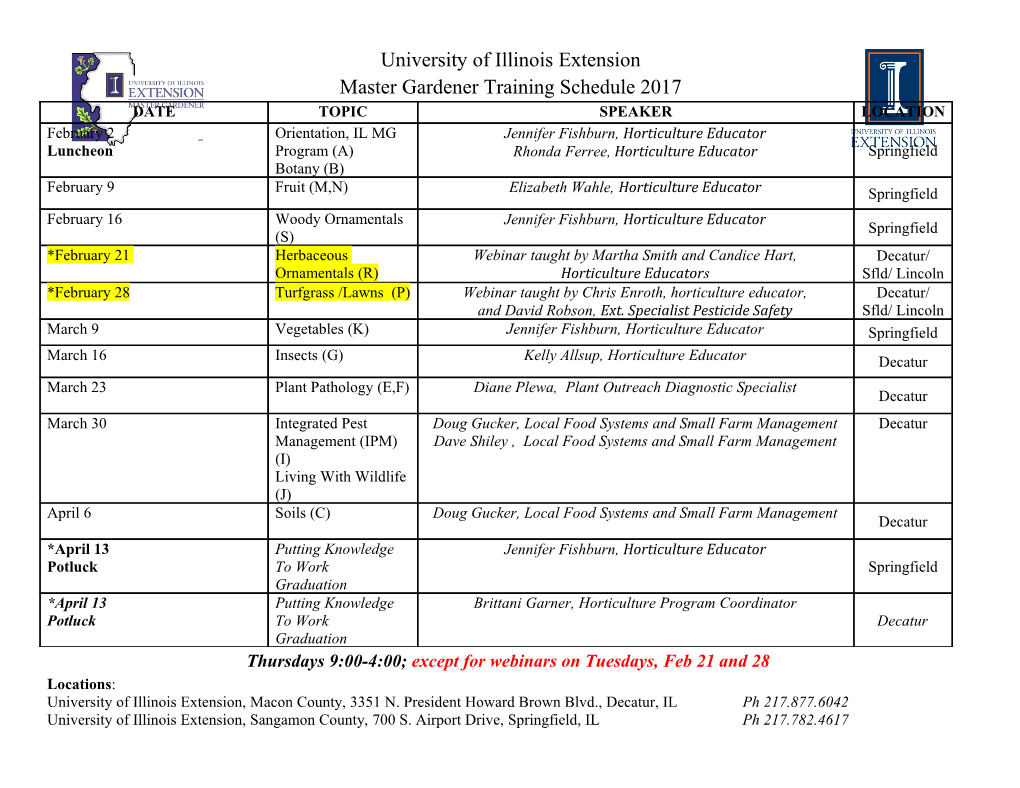
Geosci. Model Dev., 10, 2009–2030, 2017 www.geosci-model-dev.net/10/2009/2017/ doi:10.5194/gmd-10-2009-2017 © Author(s) 2017. CC Attribution 3.0 License. Carbon–nitrogen interactions in idealized simulations with JSBACH (version 3.10) Daniel S. Goll1,a, Alexander J. Winkler2,3, Thomas Raddatz2, Ning Dong3,5, Ian Colin Prentice4,6, Philippe Ciais1, and Victor Brovkin2 1Le Laboratoire des Sciences du Climat et de l’Environnement, IPSL-LSCE CEA/CNRS/UVSQ Saclay, Gif sur Yvette, France 2Max Planck Institute for Meteorology, Hamburg, Germany 3International Max Planck Research School on Earth System Modeling, Hamburg, Germany 4Department of Biological Sciences, Macquarie University, North Ryde, NSW 2109, Australia 5Faculty of Agriculture and Environment, Department of Environmental Sciences, University of Sydney, NSW 2006, Australia 6AXA Chair in Biosphere and Climate Impacts, Department of Life Sciences, Imperial College London, Silwood Park Campus, Buckhurst Road, Ascot SL5 7PY, UK aformerly at: Max Planck Institute for Meteorology, Hamburg, Germany Correspondence to: Daniel S. Goll ([email protected]) Received: 17 December 2016 – Discussion started: 9 January 2017 Revised: 7 April 2017 – Accepted: 14 April 2017 – Published: 22 May 2017 Abstract. Recent advances in the representation of soil car- The strengths of the land carbon feedbacks of the re- −1 bon decomposition and carbon–nitrogen interactions imple- cent version of JSBACH, with βL D 0:61 Pgppm and γL D mented previously into separate versions of the land sur- −27:5 Pg ◦C−1, are 34 and 53 % less than the averages of face scheme JSBACH are here combined in a single version, CMIP5 models, although the CMIP5 version of JSBACH which is set to be used in the upcoming 6th phase of coupled simulated βL and γL, which are 59 and 42 % higher than model intercomparison project (CMIP6). multi-model average. These changes are primarily due to the Here we demonstrate that the new version of JSBACH new decomposition model, indicating the importance of soil is able to reproduce the spatial variability in the reactive organic matter decomposition for land carbon feedbacks. nitrogen-loss pathways as derived from a compilation of δ15N data (R D 0:76, root mean square error (RMSE) D 0:2, Taylor score D 0:83). The inclusion of carbon–nitrogen inter- actions leads to a moderate reduction (−10 %) of the carbon- 1 Introduction concentration feedback (βL) and has a negligible effect on the sensitivity of the land carbon cycle to warming (γL) com- The version of the Max Planck Institute Earth System Model pared to the same version of the model without carbon– (MPI-ESM) used in the 5th phase of the coupled model in- nitrogen interactions in idealized simulations (1 % increase tercomparison project (CMIP5) experienced pronounced bi- in atmospheric carbon dioxide per year). In line with ev- ases in simulated soil carbon (Todd-Brown et al., 2013), idence from elevated carbon dioxide manipulation experi- soil hydrology (Hagemann and Stacke, 2014), and the lack ments, pronounced nitrogen scarcity is alleviated by (1) the of carbon–soil nutrient interactions (Zaehle et al., 2014a; accumulation of nitrogen due to enhanced nitrogen inputs by Wieder et al., 2015), hampering the reliability of the simu- biological nitrogen fixation and reduced losses by leaching lated response of land system to increasing carbon dioxide and volatilization. Warming stimulated turnover of organic (CO2), climate and land use and land cover changes. Re- nitrogen further counteracts scarcity. cent model developments addressed these issues (Goll et al., 2012, 2015; Hagemann and Stacke, 2014) in separate ver- Published by Copernicus Publications on behalf of the European Geosciences Union. 2010 D. S. Goll et al.: Carbon–nitrogen interactions in JSBACH sions of the land surface scheme of the MPI-ESM, JSBACH, coming round of CMIP experiments (Eyring et al., 2016), but have not been yet combined in a single model version. and allows for a straightforward comparison to the result The projected carbon balance in JSBACH was substan- from the previous round of CMIP (Taylor et al., 2012). tially affected by recent model developments: the implemen- tation of carbon–nitrogen–phosphorus interactions reduced accumulated land carbon uptake by 25 % between 1860– 2 Methods 2100 under a business as usual scenario (Goll et al., 2012), while the implementation of a new decomposition model 2.1 Model description (YASSO) reduced the accumulated land carbon uptake by about 60 % in the same period (Goll et al., 2015). The ex- The implementation of the nitrogen cycle and the soil car- change of the former CENTURY-type soil decomposition bon and litter decomposition model YASSO is described in model (Parton et al., 1993) with the YASSO decomposi- detail in Parida(2011), Goll et al.(2012) and Goll et al. tion model (Tuomi et al., 2008, 2009, 2011) improved the (2015), respectively. In the following, a brief summary of the present-day state of the carbon cycle compared to observa- major concepts is given and afterwards the modifications to tions as well as the response of decomposition to soil warm- the original developments needed to combine them are docu- ing, and substantially reduced the uncertainties of land use mented in detail. The notation applied here follows Goll et al. change emissions for a given land use change scenario (Goll (2012, 2015) and a scheme of the cycling of carbon and ni- et al., 2015). The strong impact on the carbon balance of each trogen as well as their interactions are given in Fig.1. of both developments underlines the importance of combin- The decomposition model (YASSO) is based on a compi- ing them in a single version. lation of litter decomposition and soil carbon data and dis- The capacity of land ecosystems to increase their nitro- tinguish organic matter fractions according to litter size and gen storage as well as to enhance recycling of nitrogen in or- solubility (Tuomi et al., 2008, 2009, 2011). In JSBACH we ganic matter are major constraints on their ability to increase use two litter size classes, which correspond to litter from carbon storage under elevated CO2 concentrations (Hungate non-lignified and lignified plant material (Goll et al., 2015). et al., 2003; Thomas et al., 2015; Liang et al., 2016). The Each of the two litter classes is further refined into four solu- respective response patterns of nitrogen processes governing bility classes (acid soluble (CA), water soluble (CW), ethanol the balance and turnover of organic nitrogen are crucial (Niu soluble (CE), non-soluble (CN)) (Eq.1). One additional pool et al., 2016) to assess the likelihood of the occurrence of (CH) represents humic, slowly decomposing substances. (progressive) nitrogen limitation (Luo et al., 2004). Recent The interactions between nitrogen availability and car- advances in the interpretation of soil δ15N global data sets bon fluxes, namely primary productivity and decomposition, provide a promising tool (Houlton et al., 2015; Zhu and Ri- are based on the concept of CO2-induced nutrient limita- ley, 2015) by allowing for a more detailed evaluation of the tion (CNL) (Goll et al., 2012). In this framework, we dis- nitrogen-loss pathways in land carbon–nitrogen models than tinguish between CNL and background nutrient limitation. previously done (e.g., Parida, 2011; Goll et al., 2012). The latter is assumed to be indirectly considered in the orig- Since future scenarios of CO2 concentrations differ among inal parametrization of carbon cycle processes as they are CMIP phases, an idealized setup of an annual increase in based on measurements in present-day ecosystems and there- CO2 concentration by 1 % is used to foster the analysis of fore reflect present-day nutrient conditions. CNL is an ad- the carbon cycle feedbacks among models, and to compare ditional nutrient limitation caused by the increase in atmo- emerging properties of different model versions in various spheric CO2 and is computed dynamically according to nutri- CMIPs (Eyring et al., 2016). We adopt this approach taking ent supply and demand. In case microbial and vegetation ni- advantage of existing simulations of climatic changes in this trogen demand cannot be met by the supply, all carbon fluxes idealized setup of the CMIP5 intercomparison (Arora et al., of which the donor compartment has a higher C : N ratio than 2013) to drive the land surface model JSBACH uncoupled the receiving pool (i.e., the fluxes of carbon from the solubil- from the atmosphere and ocean components of the Earth sys- ity classes pools to the humus pool) are downregulated. The tem model. concept of CNL allows one to introduce carbon–nitrogen in- This article documents the modifications to the soil carbon teractions to YASSO, as the needed conditions are met; e.g., decomposition (Goll et al., 2015) and nitrogen cycle (Parida, the parametrization of YASSO indirectly reflects present-day 2011; Goll et al., 2012) submodels and the combination of nutrient effects on decomposition as it is based on leaf litter both developments in a recent version of JSBACH includ- experiments. ing an advanced soil hydrological scheme (Hagemann and Following Goll et al.(2012), CNL affects the decompo- Stacke, 2014), scheduled to be used in CMIP6. We further sition of all pools except the slowly decomposing nutrient- analyzed the state of the nitrogen cycle using soil δ15N data rich pool (Eqs.2–5). The litter decomposition data on which and quantified the carbon cycle feedbacks to increasing CO2 YASSO is based is not suited to link the fate of nitrogen in concentrations and climate change. The analysis aims at fa- litter to the respective solubility pools. Therefore, we assume cilitating the interpretation of the models dynamics in the up- one single nitrogen pool representing all nitrogen linked to Geosci. Model Dev., 10, 2009–2030, 2017 www.geosci-model-dev.net/10/2009/2017/ D.
Details
-
File Typepdf
-
Upload Time-
-
Content LanguagesEnglish
-
Upload UserAnonymous/Not logged-in
-
File Pages22 Page
-
File Size-