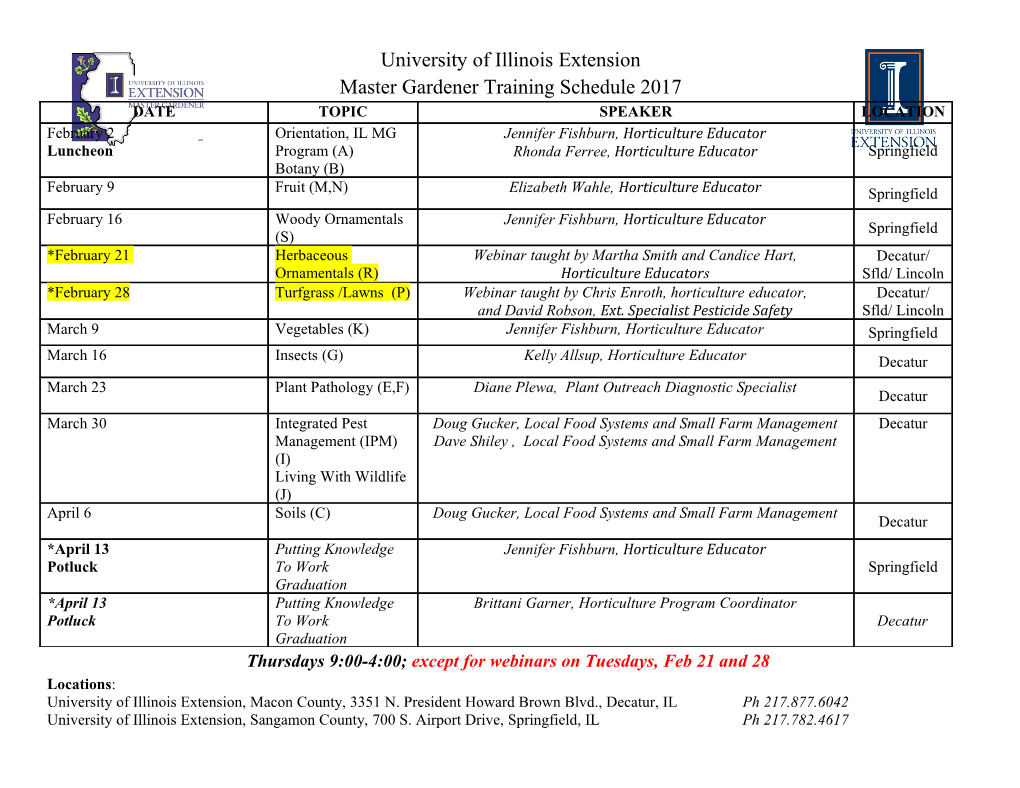
The University of Maine DigitalCommons@UMaine Honors College Spring 5-2018 Wireless Power and Data Transfer Using Inductively Resonant Coils Seth C. Raymond University of Maine Follow this and additional works at: https://digitalcommons.library.umaine.edu/honors Part of the Electrical and Computer Engineering Commons Recommended Citation Raymond, Seth C., "Wireless Power and Data Transfer Using Inductively Resonant Coils" (2018). Honors College. 428. https://digitalcommons.library.umaine.edu/honors/428 This Honors Thesis is brought to you for free and open access by DigitalCommons@UMaine. It has been accepted for inclusion in Honors College by an authorized administrator of DigitalCommons@UMaine. For more information, please contact [email protected]. WIRELESS POWER AND DATA TRANSFER USING INDUCTIVELY RESONANT COILS by Seth C. Raymond A Thesis Submitted in Partial Fulfillment of the Requirements for a Degree with Honors (Electrical Engineering) The Honors College University of Maine May 2016 Advisory Committee: Nuri Emanetoglu, Professor of Electrical and Computer Engineering, Advisor Andrew Sheaff, Professor of Electrical and Computer Engineering Donald Hummels, Professor of Electrical and Computer Engineering Elizabeth Payne, Professor of English Melissa Ladenheim, Associate Dean, Honors Abstract In electronics, resonance occurs when the admittances of circuit components, the imaginary parts of the component impedances, cancel each other out. Operation of circuits at their resonant fre- quencies can cause oscillation of signals at extreme levels. Nikola Tesla developed the concept of harnessing the capabilities of resonance by transmitting energy wirelessly between two inductively resonant coils. In 1897, his newly patented design, known as the Tesla coil, was an early prototype used to realize his theory. However, only in relatively recent history has the idea been expanded upon and used for commercial applications. Medical devices, such as pacemakers, are powered through resonant inductive coupling; this form of wireless technology has been in use since the 1960s. Battery-operated toothbrushes are typically charged with one or more small coils in the toothbrush and in the cradle, with power transmitted from the cradle to the toothbrush wirelessly. Newer emerging technology, such as radio-frequency identification (RFID) and near field commu- nication (NFC) has provided end-users with relatively simple products for proximity dependent interaction with their personal devices, such as smartphones. This paper describes the theory and limitations of this emerging technology through the design and construction of a wireless NiCd bat- tery charger, as well as through an investigation of some of the more common protocols for wireless data transmission. Contents List of Figures iv 1 Introduction 1 2 Mechanics of Wireless Transmission 1 3 Wireless Power Transfer 4 3.1 Common Wireless Power Transmission Applications . 4 3.2 Power Transmission Module . 5 3.2.1 Power Transmission Design . 6 3.2.2 Power Transmission Construction and Results . 12 3.3 Power Receiving Module . 13 3.3.1 Power Receiving Design . 13 3.3.2 Power Receiving Construction and Results . 17 4 Wireless Data Transmission 18 4.1 Near Field Communication Applications . 19 4.1.1 Application Data Transferal . 20 4.1.2 Monetary Transactions . 20 4.2 Near Field Communication Limitations . 20 5 Conclusion 21 References 22 A Schematics 23 iii List of Figures 1 Magnetic flux through Coil 2 due to current I1 ................ 3 2 Transmit module block . 6 3 Generic Wien-Bridge oscillator schematic . 7 4 Tuned Wien-Bridge oscillator with amplitude stabilization . 8 5 Final tuned Wien-Bridge oscillator schematic . 9 6 Driver circuit schematic . 10 7 Driver simulation schematic . 10 8 Driver simulation results . 11 9 Comparator circuit schematic with Class B amplifier output stage . 12 10 Receive module block . 13 11 Half-wave rectifier and voltage regulator . 14 12 Simulation schematic of half-wave rectification . 14 13 Unrectified EMF across simulated Rx coil . 15 14 Rectified EMF across simulated Rx coil . 16 15 9V NiCd battery charger . 17 16 Example of phase jitter in time domain . 19 17 Final transmitter schematic . 23 18 Final receiver schematic . 24 iv 1 Introduction Wireless power transmission has been in use since the 1960s, but with limited commercial applications. Due to the advances in this technology, increased consumer use has been seen in the last decade. One of the more prominent industries that is taking advantage of this form of wireless technology is the smartphone industry. Increasing numbers of smart- phones and wearable technology devices are integrating wireless battery charging as well as near field communication (NFC) technology. NFC is typically used to automate simple tasks, such as turning off a phone ringer, by programming what is known as a “tag.” The tag is then placed on a surface where the user will tap the phone. Once in proximity, the phone executes the programmed command. Monetary transactions can also be performed between supported devices. The intent of the addition of wireless charging and wireless data transfer is to create an easier, more intuitive user experience. The technology does, however, have its own set of limitations and restrictions. This report investigates the me- chanics of wireless power transmission and gives an elementary design of a wireless NiCd battery charging system. Research on NFC uses and limitations is also presented. Section 2 describes the electromagnetic workings of wireless transmission. Section 3 presents the design, construction, and results of a wireless battery charging system. Sec- tion 4 summarizes research on wireless data transmission used in an increasing number of smartphones. Section 5 concludes the report. 2 Mechanics of Wireless Transmission Passive circuit components include resistors, capacitors, and inductors. Each of these com- ponents have an impedance associated with them, meaning they oppose the flow of current when a voltage is applied across the terminals of the component. Impedance can be broken up into real and imaginary parts. Ideally, resistors have purely real impedances, whereas capacitors and inductors have purely imaginary impedances. The impedances of capaci- tors and inductors are frequency dependent. Frequency is represented as ! in this section. Equation 1 below describes the impedance ZL of an inductor with inductance L. ZL = j!L (1) Note that the impedance of an inductor will increase with frequency. This can be contrasted with the impedance of a capacitor. The impedance ZC of a capacitor with capacitance C is given in Equation 2. Again, the impedance of this component is frequency dependent. 1 −j Z = = (2) C j!C !C Note that with capacitors, the impedance now decreases with higher frequencies. Recalling that the resonant frequency of a circuit is the frequency at which the imaginary parts of the circuit impedance is equal to zero, it can be seen that combining capacitors and inductors 1 in some combination will result in a resonant frequency, !0, based on the inductance and capacitance of the components. A particular resonant frequency, or a tuned resonant fre- quency, can be achieved by picking inductance and capacitance values for a given circuit topology. An important note is that Equations 1 and 2 assume ideal components. Real world com- ponents are not ideal and include what are known as parasitic resistances, capacitances, and inductances, which will alter the given equations slightly and give rise to an error in the actual resonant frequency of the circuit. Component leads give rise to parasitic induc- tances. Leads and wires, such as those making up the inductive coil, produce parasitic capacitances. Finally, all wires are inherently resistive, which produces some losses. These parasitics should also be taken into account when designing resonant circuitry. With an understanding of the concept of resonance, the physical properties of inductors can now be described. Inductors inherently oppose a change in current and do so by generating a magnetic field. This field is how the inductor stores energy. In this text, vectors are represented in bold-face. The magnetic field, B, at a distance r away from a straight wire with current I flowing through it is described in Equation 3. The permeability of free space, −7 −2 µ0, is constant and is taken as 4π × 10 [NA ]. µ I B = 0 (3) 2πr An inductor can be described as a wire wound about an axis. The magnetic field produced by the inductor is now focused in the direction of the axis about which the wire is wrapped. The magnetic field produced by an inductor with N turns and length ` is given in Equation 4. µ IN B = 0 (4) ` Again, inductors can be used to store energy in a circuit. When the current through an inductor increases or decreases, the magnetic field produced by the inductor will either expand or collapse respectively. This change in magnetic field ideally keeps the number of electrons flowing through the inductor constant by either extracting energy from the inductor or returning energy to it. The change in current also results in a change in voltage across the inductor. Through electromotive self-induction, the change in strength of a magnetic field produced by an inductor gives rise to a corresponding voltage across the inductor. This electromotive force (EMF) has a polarity that opposes the change of current. The EMF across an inductor with dI inductance L and time-changing current dt is defined in Equation 5. dI " = L (5) dt With the knowledge of how energy is stored in an inductor, a related parameter can now be described. The magnetic flux is defined as the amount of magnetic field passing through a 2 given area. Magnetic flux, Φ, is mathematically defined in Equation 6. Φ = B · A = jBjjAjcos(θ) (6) It should be noted that the vector area of the surface A has a magnitude equal to the surface area of the object and points normal to the surface. The value of θ is defined as the angle between B and A.
Details
-
File Typepdf
-
Upload Time-
-
Content LanguagesEnglish
-
Upload UserAnonymous/Not logged-in
-
File Pages30 Page
-
File Size-