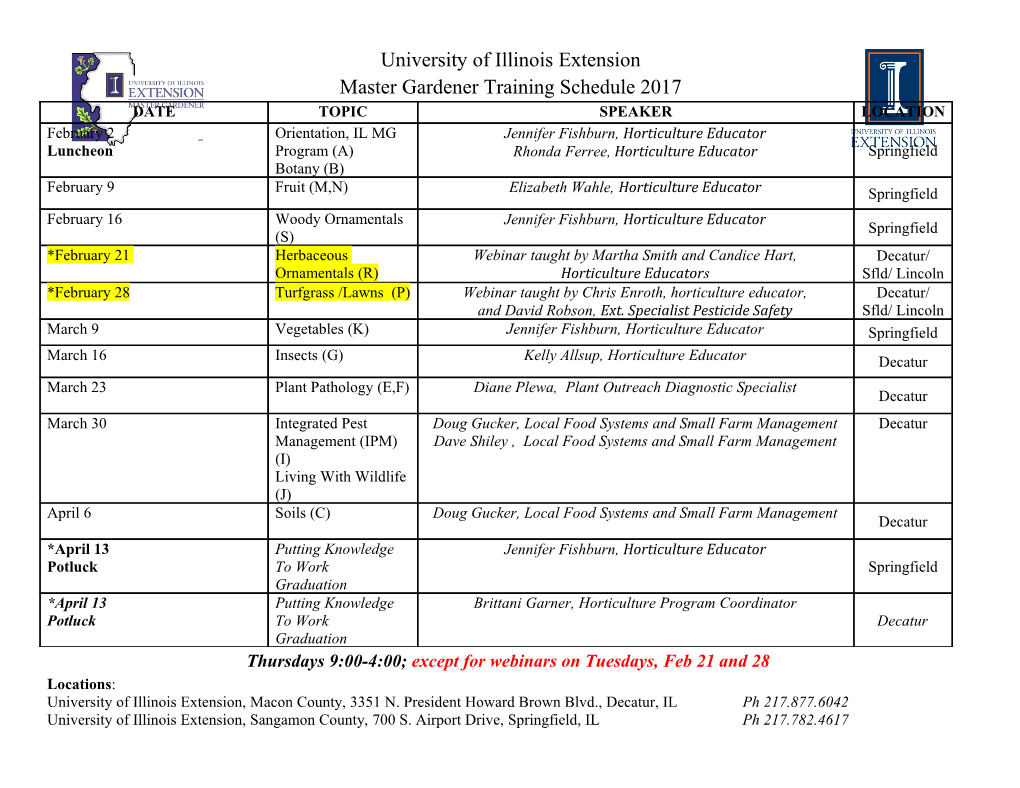
23-1 / H. Baur Active Matrix Technologies for AMLCD and AMOLED Application Holger Baur*, Thomas Buergstein, Silke Goettling, Rene Hlawatsch, Sven Jelting, Efstathios Persidis, Fabio Pieralisi, Patrick Schalberger, Axel Schindler Norbert Fruehauf Chair of Display Technology, University of Stuttgart, Stuttgart, Germany Phone: +49 711 685 66922, E-mail: [email protected] Abstract used for reducing the costs for AMOLED display The Chair of Display Technology at the University production. Topgate μc-Si TFTs are more of Stuttgart develops various technologies for active complicated to manufacture but show higher carrier matrix applications. Last year we presented an LTPS mobilities than the bottomgate devices and the effort active matrix process without the need for ion is still less than for LTPS production. In the implantation. This process is compared to other AM meanwhile electron mobilities up to 150cm²/Vs are processes and the technological demands for different reported [3], but the devices still suffer from high applications are discussed. leakage currents. This process might have the potential to replace LTPS in some applications. 1. Introduction For the application in flexible displays especially in Different display applications require different combination with bistable display effects TFTs technologies for realizing the active matrix. Often realized with organic semiconducting materials have there is a trade off between requirements, technical gained more and more attention recently. For really possibilities and production cost to determine which flexible displays conventional inorganic TFT process is suitable and applicable for the desired technologies like a-Si are not well suited because of display application and production. the different thermal, mechanical and structural Low temperature poly silicon (LTPS) technology is properties compared to plastic substrates. Active widely accepted for AMOLED displays [1]. Due to matrix displays with a-Si TFTs on plastic substrates the two orders of magnitude higher carrier mobility have been realized in the past [4] but for the compared to amorphous silicon (a-Si), LTPS thin film mentioned reasons display sizes and bendability are transistors (TFTs) can provide much higher current limited. However with organic TFTs even rollable than a-Si TFTs. For this reason and because of the displays have been demonstrated in the meanwhile better device stability (eg. threshold voltage shift and [5]. output current stability) under DC operation LTPS is preferred for AMOLED application. Drawback of We have developed simple and cost effective LTPS LTPS technology is the more complicated topgate processes for active matrix display applications. The process, the need for extra equipment for excimer NMOS and PMOS processes were proven by laser annealing (ELA) and ion implantation and the manufactured display demonstrators. With the CMOS limitation of substrate sizes. This makes the process process TFTs, inverters, ring oscillators and shift expensive and incompatible with todays a-Si registers have been realized. production lines. An additional advantage of LTPS For future flexible displays we are investigating compared to a-Si is the availability of CMOS alternative technologies for realizing TFTs and active technology that enables the integration of high matrixes on flexible substrates. Promising performance driving circuitry to eliminate external technologies are microcrystalline silicon for drivers and to save costs for AMOLED production. AMOLED displays and organic or carbon nanotube Microcrystalline silicon (μc-Si) directly deposited by TFTs for LCD or electrophoretic displays based on plasma enhanced chemical vapor deposition active matrixes. (PECVD) is a promising material for cheaper active matrix display manufacturing. Bottom gate μc-Si TFTs show electron mobilites slightly higher than a- Si but are stable under DC operation [2]. This process is compatible with a-Si production lines and could be IMID/IDMC '06 DIGEST • 451 23-1 / H. Baur 2. Results We implemented a three TFT p-mos pixel circuit which provides the possibility for testing the active 2.1 Self aligned implanted LTPS TFT process matrix prior to the OLED deposition. This circuit For AMOLED application we have developed a four enables additionally the measurement of OLED mask LTPS TFT process with self aligned formation forward voltages at given currents to compensate for of the drain and source areas by ion implantation. The the aging during the lifetime of the display. The process flow is as follows: OLED depositions and the back end were performed First a buffer layer of SiO2 and an intrinsic a-Si layer by project partners within the OLEDFAB consortium are deposited by PECVD. After dehydrogenation [7]. A photograph of one of the realized single area excimer laser crystallization (SAELC) [6] demonstrators is shown in Figure 1. The distorted is performed. The semiconductor islands are defined columns in the left part of the display are caused by with the first photolithography step and plasma failed bond contacts of one of the column drivers. etching. The gateoxide is deposited by PECVD and after sputter depositing the gate metal the gate electrodes are defined with the second mask step and 2.2 LTPS TFT process with deposited drain/source wet chemical etching. Drain and source areas are areas created by self aligned ion implantation and a second The self aligned implanted 4 mask LTPS TFT process excimer laser treatment for dopant activation. The works well for p-channel TFTs where no low doped interlayer dielectric, that acts as passivation as well, is drain (LDD) is required. Implementing additional deposited by PECVD and the third mask is used to LDD structures for improved n-channel operation define the vias that are opened by reactive ion etching increases the number of implantation steps and, (RIE). After sputter depositing the drain/source metal dependent on the process, even the number of the fourth mask step for patterning the contacts required masks [8]. This is raising the production completes the TFT process. costs considerably. Furthermore ion implantation equipment is among the most costly equipment for Average TFTs built with this process show a LTPS backplane manufacturing and a limiting factor p-channel mobility of 40cm²/Vs, an on/off ratio of for substrate sizes. For these reasons we have more than 5*106 and a subthreshold slope of developed an LTPS active matrix process with 750mV/dec. PECVD doped drain/source contacts [9]. The Based on this process we have developed and realized implantation free low temperature poly silicon TFT active matrix backplanes for full color 4.4 inch qVGA process is illustrated in Figure 2. A PECVD deposited OLED displays [7]. layer is used as dopant source. The substitution of ion doping by PECVD deposition overcomes a major limitation for panel sizes in poly-Si technology and avoids large investment costs for ion implantation equipment. First a highly doped silicon layer is deposited by PECVD. The doped silicon is structured by photolithography and plasma etching to form the drain and source region of the TFT in a later process step (a). After that the intrinsic silicon layer is deposited (b). The two PECVD processes were adapted to each other, in order not to incorporate the phosphorous to the TFT channel by etchback of the doped silicon during the deposition of the intrinsic silicon. After dehydrogenation the amorphous silicon Figure 1: 4.4’’ qVGA AMOLED display is crystallized with an area excimer laser and is demonstrator (monochrome blue) structured to islands (c). Within the laser crystallization step the dopant atoms diffuse to the 452 • IMID/IDMC '06 DIGEST 23-1 / H. Baur overlaying silicon, are activated and form the drain is difficult to anneal completely in a self aligned and source region. implanted TFT process [11] is avoided as well. After PECVD deposition of the gateoxide the gate 240 metallization is sputter deposited and structured wet TFTs spaced 5mm 220 chemically (d). Because of the non self aligned 10 pulses per area 200 ambient atmoshpere process the gate has to overlap drain and source to 180 avoid an offset region by misalignment during the 160 [cm²/Vs] photolithography. e 140 120 To complete the TFTs, the interlayer dielectric is 100 deposited (e), via holes are opened and the contact 80 metallization is sputter deposited and structured (f). 60 40 mean value and standard deviation n+-Si μ Mobility Electron 20 of 5 TFTs Bufferoxide 0 520 540 560 580 600 620 640 Substrate a) Laser Energy Density [mJ/cm²] a-Si Bufferoxide Figure 3: Electron mobility versus laser energy Substrate density b) poly-Si Drain Source Bufferoxide For application as switching TFTs in AMLCD or Substrate AMOLED displays we realized TFTs with a channel c) geometry of 20μm*20μm with and without doped Gate region in the channel. The selectively doped region Gateoxide Drain Source Bufferoxide (SDR) reduces the off-current by lowering the Substrate electrical field at the drain junction in the off-state d) without reducing the drain current in the on-state [12]. With this method we realized TFTs with an electron Interlayer 7 Gateoxide Drain Source mobility of μe=200cm²/Vs and an on/off ratio > 10 . Bufferoxide Input characteristics of TFTs with/without SDR are Substrate shown in Figure 4. e) 1m Interlayer Drain Source V =10V Gateoxide 100μ DS Bufferoxide Substrate 10μ f) 1μ Figure 2: Process flow for poly-Si TFTs with 100n PECVD doped drain/source areas [A] DS I 10n SDR TFT With this process we were able to fabricate operating 1n W*L=(20*10)μm²+(20*10)μm² standard TFT TFTs over a 120mJ/cm² wide range of laser energy 100p densities. The process allows the fabrication of TFTs W*L=(20*20)μm² 10p with different electron mobilities for active matrix and -10 -5 0 5 10 15 20 V [V] driving electronics integration on the same panel. GS Dependent on the applied laser energy density TFTs with electron mobilities of μe=20cm²/Vs (at Figure 4: Input characteristic of TFTs 520mJ/cm²) up to μe=200cm²/Vs (at 640mJ/cm²) were with/without SDR processed on the same glass substrate (see Figure 3).
Details
-
File Typepdf
-
Upload Time-
-
Content LanguagesEnglish
-
Upload UserAnonymous/Not logged-in
-
File Pages8 Page
-
File Size-