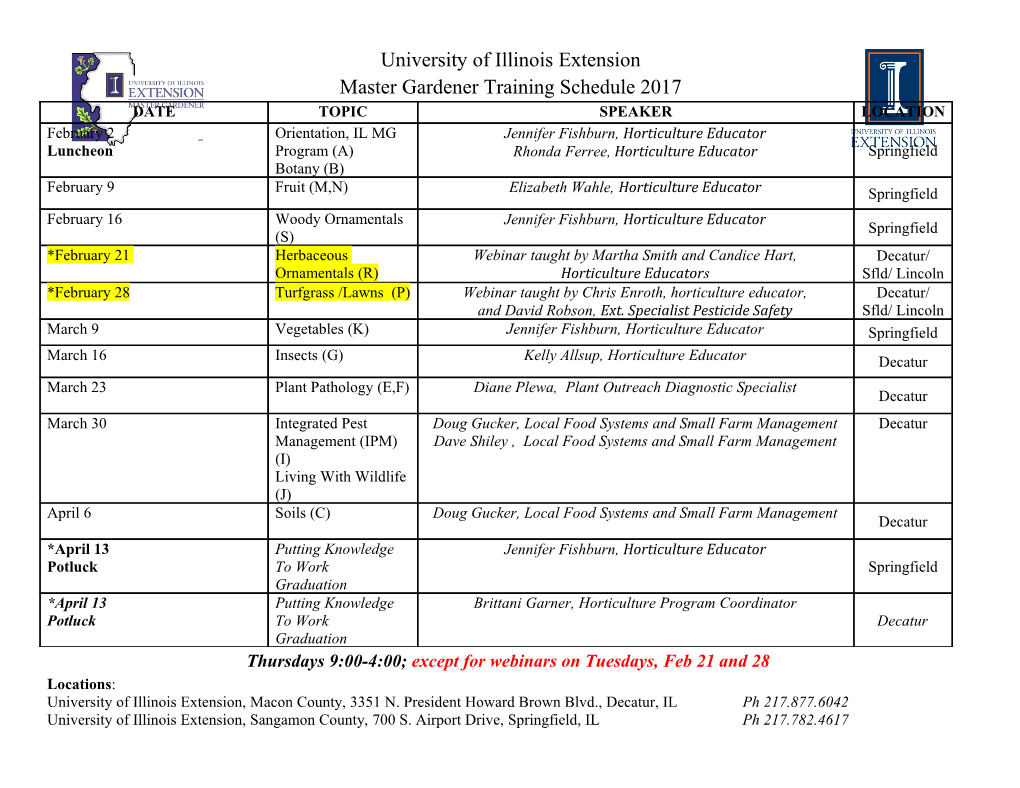
Theoretical Ecology https://doi.org/10.1007/s12080-019-0423-6 ORIGINAL PAPER Ecological determinants of pathogen transmission in communally roosting species Andrew J. Laughlin1 & Richard J. Hall2,3,4 & Caz M. Taylor5 Received: 16 July 2018 /Accepted: 6 March 2019 # Springer Nature B.V. 2019 Abstract Many animals derive benefits from roosting communally but may also face increased risk of infectious disease transmission. In spite of recent high-profile disease outbreaks in roosting animals of conservation and public health concern, we currently lack general theory for how attributes of roosting animals and their pathogens influence pathogen spread among roosts and overall population impacts on roosting species. Here we develop a model to explore how roost size and host site fidelity influence the time for a pathogen to escape from its initial roost, overall infection prevalence, and host population size, for pathogens with density- or frequency-dependent transmission and varying virulence. We find that pathogens spread rapidly to all roosts when animals are distributed among a small number of large roosts, and that roost size more strongly influences spread rate for density- dependent than frequency-dependent transmitted pathogens. However, roosting animals that exhibit high site fidelity and dis- tribute among a large number of small roosts are buffered from population-level impacts of pathogens of both transmission modes. We discuss our results in the context of anthropogenic change that is altering aspects of roosting behavior relevant to emerging pathogen spread. Keywords Animal aggregations . Communal roost . Pathogen transmission . Roost size . Site fidelity Introduction Hamilton 1982; Thompson 1989), fish (Clough and Ladle, 1997), birds (Eiserer 1984; Beauchamp 1999), and inverte- Animal aggregations occur in a wide variety of taxa, at vary- brates (Mallet 1986;GretherandSwitzer2000). Roosts can ing spatial and temporal scales, and represent some of the range in size from just a few individuals to spectacular aggre- most striking patterns of emergent behavior in natural systems gations of millions of animals. Roosts vary substantially with- (Parrish and Edelstein-Keshet 1999). Communal roosts, here in and among species in attributes including their duration and defined as aggregations of conspecifics during the inactive seasonality (Eiserer 1984; O'Shea and Bogan, 2003; Kunz period of the diurnal cycle (Grether et al. 2014), have been 1982), spatial reliability of their location (Yadon 1956; documented in many species of mammals (Kunz 1982; O’Donnell and Sedgeley 1999), and the degree to which indi- viduals intermix between roosts to form Broost systems^ (Leyrer et al. 2006; Grether and Switzer 2000; Laughlin * Andrew J. Laughlin et al. 2014). Given that roosts provide important ecological [email protected] functions such as Bnutrient hotspots^ from guano (Duchamp et al. 2010) and ecosystem services such as pest control 1 Department of Environmental Studies, University of North Carolina (Cleveland et al. 2006), understanding how these roost attri- at Asheville, Asheville, NC, USA butesinfluencethepopulationdynamicsofroostingspecies, 2 Odum School of Ecology, University of Georgia, Athens, GA, USA and their ecological interactions, is a question of practical 3 Department of Infectious Diseases, College of Veterinary Medicine, importance. University of Georgia, Athens, GA, USA In previous work, we explored how different roost dynam- 4 Center for the Ecology of Infectious Diseases, University of Georgia, ics can emerge from different aggregative behaviors (Laughlin Athens, GA, USA et al. 2014). By changing the strengths of roost-site fidelity 5 Department of Ecology and Evolutionary Biology, Tulane (the tendency for an individual to return to its previous roost) University, New Orleans, LA, USA and conspecific attraction (the tendency for an individual to Theor Ecol group together with conspecifics), different roost systems as large caves, could result in density-dependent transmission. emerged including systems with many small roosts and low Additionally, the daily rate of movement between roost sites (a individual fidelity, few large roosts and high fidelity to them, function of individual site fidelity and roost size) will deter- and several systems in between these extremes. Roost dynam- mine rate of spatial spread within a roost network. While ics can thus be more simply described in two dimensions: the models have been developed to explore drivers of disease in size of the roosts and site fidelity. focal host-pathogen systems (Plowright et al. 2011; Maher Communal roosting can provide benefits such as decreased et al. 2012), to date we lack general theory about how attri- individual predation risk (Hamilton 1971), information shar- butes of roosts (e.g., size), hosts (e.g., site fidelity), and path- ing (Ward and Zahavi 1973), and thermoregulation (Eiserer ogens (e.g., transmission mode and virulence) interact to de- 1984), but these aggregations can also lead to negative out- termine pathogen impacts and spatial spread within a network comes such as facilitating transmission of pathogens and par- of roosts. asites (Krause and Ruxton 2002; Altizer et al. 2006). For In this study, we developed simple deterministic and example, Phocine distemper virus is spread among Harbor stochastic models to explore different aspects of pathogen seals (Phoca vitulina) at their haulouts where seals aggregate dynamics in communally roosting host species. We devel- seasonally on sea ice or beaches (Swinton et al. 1998), occa- oped an aggregated deterministic model in which we cal- sionally leading to large outbreaks. Moreover, roosts have culated the time it took for the disease to escape a roost been implicated as transmission hubs for emerging pathogens under different roost size and fidelity rate combinations, that cause mass mortality of their hosts, as observed in North and under different pathogen transmission modes. American songbirds following the introduction of West Nile Because time and population size are treated as continu- virus (Diuk-Wasser et al. 2010;Dawsonetal.2007)andin ous variables in the deterministic model and because such cave-roosting bats infected with the fungus causing White- models are known to not accurately predict dynamics in nose syndrome (Frick et al. 2010; Langwig et al. 2015; small populations, we developed a stochastic individual- Lorch et al. 2013). based model to further explore the effects of, and interac- Human activity can profoundly alter local and regional tions between, population size, roost size, roost-site fidel- dynamics of roosting species with consequences for infection ity, pathogen transmission mode, and virulence on the risk. Human-made structures could provide new roosting hab- spread of an infectious disease through a population. itats or create barriers to inter-roost movement. Food subsidies The modeling approach we adopt here is structurally sim- in the form of crops and exotic plantings could increase the ilar to other models of disease spread among patches duration of roost site occupancy, roost size, and site fidelity of linked by dispersal (e.g., Arino et al. 2005, McCormack individuals, increasing the likelihood of spillover to domestic and Allen 2007), which are usually applied over multiple animals and humans. For example, flying foxes (Pteropus host generations to determine conditions for endemic per- spp.) in SE Asia are attracted to palm oil cultivation, and sistence of pathogens. In our analysis, however, we quan- contact with their feces is causing human cases of Nipah virus tify the time until the pathogen establishes in a second with high fatality (Deka & Morshed 2018). The formation of roost and the effect of the disease on seasonal survival semi-permanent large roosts of flying foxes in urban and sub- as well as infection prevalence at the end of the nonbreed- urban Australia has led to local outbreaks of Hendra virus in ing season in order to focus on understanding how roost horses and humans (Plowright et al. 2011) and has led to calls size and roosting behavior affects the transient dynamics for these roosts to be forcibly removed. Understanding the of pathogen colonization following introduction during a consequences of anthropogenic change in roosting behavior single nonbreeding season. is thus a pressing question of public health and conservation concern. Standard epidemic theory suggests that locally, within a Deterministic model roost system, roost size could influence pathogen invasion, as well as the size and duration of a local epidemic. How roost Model development size relates to pathogen invasion and persistence depends crit- ically on the transmission mode. Importantly, attributes of Here we develop a model for a roosting species during a roosting behavior and/or roost structure could alter contact nonbreeding period, and for simplicity assume that non- structure for close contact-transmitted pathogens. For exam- breeding mortality during this period is negligible in the ple, roosting structures such as trees could distribute animals absence of infection. The total population (size N) distrib- into smaller sub-groups leading to frequency-dependent trans- utes roughly equally among r roosts,sothatintheab- mission, in which the probability of a susceptible becoming sence of infection, the expected population size in each infected is independent of total roost size, whereas roosts roost is Nr = N/r. All individuals of the roosting species where
Details
-
File Typepdf
-
Upload Time-
-
Content LanguagesEnglish
-
Upload UserAnonymous/Not logged-in
-
File Pages11 Page
-
File Size-