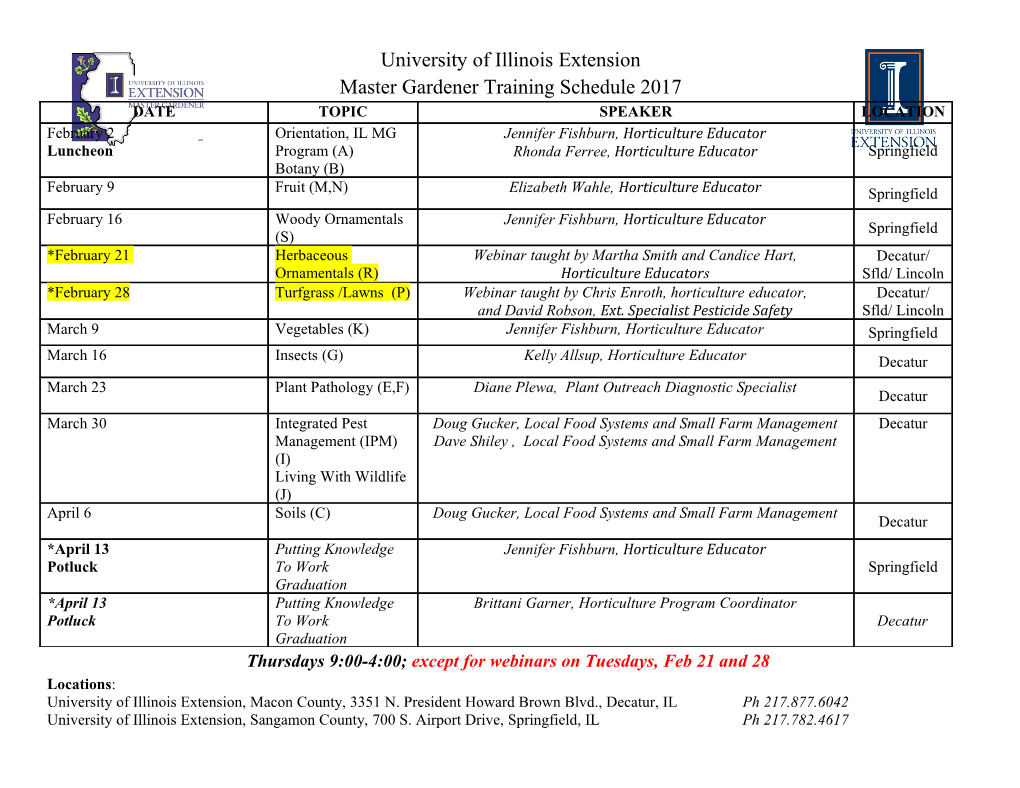
47th Lunar and Planetary Science Conference (2016) 2864.pdf THE NEUTRAL ATMOSPHERES OF PLUTO AND CHARON. M. E. Summers1, G. R. Gladstone2,3, S. A. Stern4, K. Ennico5, C. B. Olkin4, H. A. Weaver5, L. A. Young4, D. F. Strobel7, D. P. Hinson8, J. A. Kammer4, A. H. Parker4, A. J. Steffl4, I. R. Linscott6, J. Wm. Parker4, A. F. Cheng7, C. M. Lisse7, M. H. Versteeg2, T. K. Greathouse2, K. D. Retherford2,3, W. W. Woods6, K. N. Singer4, C. C. C. Tsang4, E. Schindhelm4, M. L. Wong8, Y. L. Yung8, X. Zhu7, P. Lavvas9, M. Ewell1, A. D. Jacobs1, and G. L. Tyler6, and the New Horizons Atmospheres Team. 1George Mason University, Fairfax, VA 22030, [email protected], 2Southwest Research Institute, San Antonio, TX 78238, 3The University of Texas at San Antonio, San Antonio, TX 78238, 4Southwest Research Insti- tute, Boulder, CO 80302, 5National Aeronautics and Space Administration, Ames Research Center, Space Science Division, Moffett Field, CA 94035, 6Stanford University, Palo Alto, CA, 7The Johns Hopkins University Applied Physics Laboratory, Laurel, MD 20723, 8California Institute of Technology, Pasadena, CA 91125, 9Groupe de Spec- troscopie Moléculaire et Atmosphérique, Université Reims Champagne-Ardenne, 51687 Reims, France. Introduction: The New Horizons flyby of Pluto colder upper atmosphere means that escape occurs via has given us the first close view of its surface and neu- thermal Jeans evaporation rather than the hydrodynam- tral atmosphere. The flyby observations revealed a ic outflow mechanism that was widely predicted before surprisingly complex atmosphere, with a completely encounter. Inferred escape rates based upon modeling unexpected radial temperature (and thus density) struc- the more compact atmosphere are two to three orders ture, as well as numerous haze layers in the region of of magnitude lower than pre-encounter predictions. the atmosphere where they were least expected [1]. The Alice solar occultation also revealed absorp- These observations have allow us to develop a picture tion by methane at high altitudes, consistent with a (albeit tentative) of the processes which control the methane near-surface mixing ratio of ~0.008. But me- atmosphere’s overall structure and composition. The thane appears to be the dominant constituent near the New Horizons observations have also given us some exobase. Acetylene and ethylene were observed with intriguing hints as to how the atmosphere may evolve mixing ratios in the range 1 - 4 x 10-4 between 250 and on seasonal and perhaps much longer timescales. 600 km altitude. These values imply weak vertical The complement of instruments on the New Hori- eddy mixing in the atmosphere, with a turbosphere zons spacecraft included 1) a radio science experiment confined to the below 200 km altitude. (REX) which provided near-surface pressure and tem- A global atmospheric haze was observed to extend perature profiles and ionospheric detection cabability, to altitudes at least 200 km, with at least 20 individual 2) an ultraviolet spectrograph (Alice) that gave infor- imbedded, thin (~3-5 km, or less), layers as illustrated mation on atmospheric composition, temperature and in Figure 1. A lower atmospheric haze was expected hazes, and 3) imagers (LORRI and MVIC) that provid- from prior stellar occultations, but had been inferred to ed images of Pluto’s haze layers at a range of phase exist in only in the lowest 20 km where photochemical angles, as well as of surface volatiles. Plasma instru- products such as hydrocarbons and nitriles would con- ments aboard New Horizons yielded other information dense at the low temperatures in this region of the at- not discussed here. mosphere. The lowest of the observed haze layers (~6- Pluto’s Atmosphere: The REX instrument directly 10 km altitude) extends horizontally over more than a measured Pluto’s surface pressure at the ingress and thousand kilometers. This lowest layer may be the pre- egress points during the Earth-spacecraft radio occulta- dicted photochemical haze. tion of Pluto’s atmosphere. The ingress and egress surface pressure determinations were consistent at ~10 microbar, and revealed a strong temperature inversion in the lower scale height of the atmosphere, consistent with atmospheric temperature profiles retrieved from prior Earth-based stellar occultation measurements. The Alice experiment observed nitrogen opacity in solar occultation to altitudes near ~1300 km, with again virtually symmetric profiles for ingress/egress. The nitrogen opacity at high altitudes was much lower than pre-encounter expectations, implying a much colder, and thus more compact upper atmosphere [2]. Figure 1. Image of Pluto’s limb, showing solar backlit The lower termperatures argue for an additional cool- haze with thin embedded layers. ing agent in thermodynamic models, perhaps HCN Pluto’s haze may be analogous to Titan’s detached (recently detected by ALMA observations [3]). The haze layer [4], which occurs at roughly the same pres- 47th Lunar and Planetary Science Conference (2016) 2864.pdf sure level at Pluto’s. The formation of Titan’s haze is (~ 10 km) is about 7 days, or comparable to Pluto’s 6.4 thought to be initiated by nucleation via electron- day rotation rate. The timescale for vertical dispersal attachment to hydrocarbons at ionospheric heights of the thin layers, due to different size particles falling producing extended tholin chains. Sedimentation of at different velocities, is thought to be very short, rang- these small nuclei is accompanied initially by diffu- ing from hours to days. The growth time scale for 0.1 sional growth, and later by growth due to coagulation. micron particles, however, ranges from one to several Our new ionospheric calculations (incorporationg days. This argues for a monodispersive distribution of the colder and more compact upper atmosphere) show particles in the layers, as well as a rapid formation that the ionosphere peak is ~700-900 km altitude, sig- mechanism, a diurnal production modulation, and a nificantly above the observed haze. Diffusional growth wrapping of the layers around the planet as they fall. as the particles fall will lead to near-spherical nuclei at This would require standing waves of supersaturation high altitudes above 200 km. But upon falling through with similar propagation timescales. the haze layer, growth by coagulation may lead to Charon’s Atmosphere: Neither the REX radio more complex structures and perhaps fractals. As the occultation nor the Alice solar occultation detected an fractal particles fall through the lowest photochemical atmosphere on Charon, with an 0.1 nanobar upper limit haze layer, the particles may add a coating of hydro- on surface pressure [5]. Pluto’s low atmospheric es- carbons and nitriles. cape rate (due to the cold upper atmosphere) is con- The thin embedded haze layers are much more nu- sistent with the non-detection of an atmosphere on merous, and thinner, than the analogous thin layers Charon. However, Charon’s dark pole invites specula- observed in Titan’s detached layer (typically 2-4 lay- tion that its formation could be chemical alteration of ers). Understanding their formation for both Pluto and cold polar surfaces by chemical reactions involving Titan is problematic because of their existence in a adsorbed atoms, molecules or radicals gravitationally region of the atmosphere that is highly subsaturated. captured from the gas streaming away from Pluto. Buoyancy waves generated by slow winds flowing It is important to note that the current escape rate of over mountains may produce propogating (or even Pluto may not be representative of a long-term Pluto perhaps standing) temperature waves that have suffi- average. Understanding the distribution of volatiles on ciently large amplitudes to produce narrow vertical Pluto’s surface and how they are influenced by climat- layers of supersaturation. The highly uncertain satura- ic variation might help us understand how Pluto’s at- tion vapor pressures of the various hydrocarbons and mospheric escape rate may have been different in the nitriles at the low temperature encountered in Pluto’s past. Climatic change on Pluto could also change the atmosphere makes accurate calculations of saturation climate and surface chemistry on Charon, as well as values impossible. However, the range of uncertainties how much atmosphere it has. allow the possibility that HCN could be the condensate Conclusions: The New Horizons observations making up the individual thin layers. have delivered a revolution in our understanding of Pluto’s atmosphere, and have set stringent constraints on Charon’s atmosphere. These observations have also revealed some major surprises, such as the unexpected- ly cold upper atmosphere of Pluto, and the globally extensive haze with its numerous thin embedded lay- ers. These results suggest that an unknown agent, per- haps HCN, may play a larger role in atmospheric chemistry and thermodynamic processes than previ- ously expected. The more compact upper atmosphere also implies drastically lower escape rates of volatiles from Pluto than predicted, and so has important impli- cations for volatile recycling and the long term evolu- Figure 2. This shows Pluto’s atmospheric model tem- tion of Pluto’s atmosphere. perature profile (bottom scale) and particle sedimenta- References: [1] S. A. Stern, et al. (2015) Science, tion time (top scale). 350, (2015). [2] L. A. Young et al. (2007) Space Sci- ence Review, 140, 93-127. [3] Lellouch Gurwell et al. Sedimentation timescales of the haze layer parti- 2015 AAS/DPS.4710507L). [4] P. Lavvas et al. (2013) cles range from days to months. Interestingly, the PNAS, 110, 2729-2734. [5] Gladstone et al. (2015), timescale for 0.1 micron particles at 30 km altitude to AAS/DPS471115G fall the average distance between observed haze layers .
Details
-
File Typepdf
-
Upload Time-
-
Content LanguagesEnglish
-
Upload UserAnonymous/Not logged-in
-
File Pages2 Page
-
File Size-