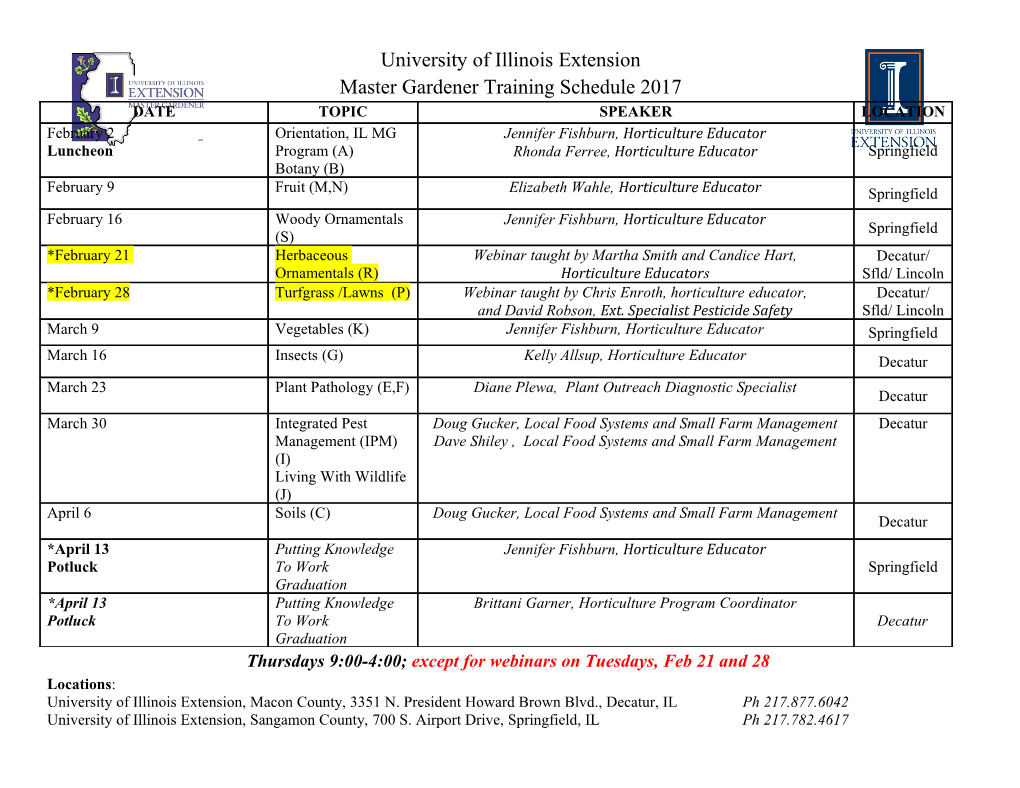
Neuron, Vol. 15, 45-54, July, 1995, Copynght 0 1995 by Cell Press Subdivision of the Drosophila Mushroom Bodies by Enhancer-Trap Expression Patterns Ming Yao Yang,‘S J. Douglas Armstrong,‘* (Heisenberg et al., 1985; Heisenberg, 1989). Visual asso- llya Vilinsky,t Nicholas J. Strausfeld,t ciative behavior and other aspects of brain anatomy ap- and Kim Kaiser* pear relatively normal. Olfactory learning is even more *Laboratory of Genetics profoundly affected by ablation of mushroom body neuro- University of Glasgow blasts at an early stage of development, depleting the adult Church Street brain of mushroom body intrinsic neurons (deBelle and Glasgow Gil 5JS Heisenberg, 1994). Additional support for a role of Dro- Scotland sophila mushroom bodies in olfactory learning derives tArizona Research Laboratories from studies of biochemical learning mutants. Expression Division of Neurobiology of the learning genes dunce and rutabaga, for example, University of Arizona occurs predominantly, though not exclusively, in the Tucson, Arizona 85721 mushroom bodies (Nighorn et al., 1991; Han et al., 1992; Davis, 1993). Finally, gynandromorph analysis implicates Drosophila mushroom bodies, or adjacent neuropils, in Summary control of the male courtship repertoire (Hall, 1979), a be- havior that relies heavily on olfaction. Taken together, the Phylogenetically conserved brain centers known as picture that emerges is of a specialized neuropil involved mushroom bodies are implicated in insect associative in associating and storing multimodal sensory information, learning and in several other aspects of insect behav- thereby providing the organism with memory and pre- ior. Kenyon cells, the intrinsic neurons of mushroom dictive behavior. bodies, have been generally considered to be dis- Mushroom bodies comprise three classes of neurons: posed as homogenous arrays. Such a simple picture input, output, and intrinsic. Both structural (Strausfeld, imposesconstraintson interpreting the diverse behav- 1976; Mobbs, 1982) and functional (Mauelshagen, 1993; ioral and computational properties that mushroom Mizunami et al., 1993; Laurent and Davidowitz, 1994) stud- bodies are supposed to perform. Using a P[GAL4] en- ies suggest that the fundamental computational properties hancer-trap approach, we have revealed axonal pro- of mushroom bodies are provided by intrinsic neurons. cesses corresponding to intrinsic cells of the Drosoph- Known as Kenyon cells, these arise from dense clusters of ila mushroom bodies. Rather than being homogenous, cell bodies dorsal and posterior to each brain hemisphere we find the Drosophila mushroom bodies to be com- (Figure 1, top). Their dendrites form the mushroom body pound neuropils in which parallel subcomponents ex- calyces, large regions of input from the olfactory lobes, hibit discrete patterns of gene expression. Different while beneath each calyx the Kenyon cell axons converge patterns correspond to hitherto unobserved differ- to form a stalk-like structure, the pedunculus. The pedun- ences in Kenyon cell trajectory and placement. On the culus extends almost to the front of the brain, at which basis of this unexpected complexity, we propose a point it gives rise to a number of lobes: a dorsally projecting model for mushroom body function in which parallel a lobe and a 8/y lobe complex projecting toward the mid- channels of information flow, perhaps with different line. Though present in Drosophila and Lepidoptera (Pear- computational properties, subserve different behav- son, 1971), the y component has not been described for ioral roles. all insects. Mushroom bodies have often been considered as iso- Introduction morphic arrays of functionally equivalent intrinsic neurons (Hanstrom, 1928; Laurent and Davidowitz, 1994). Among Insect brains contain a pair of prominent and characteristi- the properties hypothesized for an isomorphic array of cally shaped neural centers known as mushroom bodies Kenyon cells are acquired tuning to a specific odorant (Dujardin, 1850; Mobbs, 1982; Schiirmann, 1987; Straus- (Heisenberg, 1989; Laurent and Davidowitz, 1994) and feld et al., 1995). Mushroom bodies have been implicated selective strengthening of specific connections during as- in associative learning and memory, and in a variety of sociative memory(Erber et al., 1980; Mauelshagen, 1993). complex functions including courtship, motor control, and There is, on the other hand, some evidence to suggest spatial recognition and target location (Huber, 1960; Erber that mushroom bodies are not entirely isomorphic. Three et al., 1987; Mizunami et al., 1993). In the fruitfly Drosoph- Kenyon cell populations have been described for the bee, ila melanogaster, single gene mutations that cause defec- differing in terms of dendritic morphologies, calycal loca- tive mushroom bodyanatomies(e.g.,mushroombodymin- tions, and sensory input (Mobbs, 1982; Menzel et al., iature and mushroom bodies deranged) have been shown 1994) while bee lobes show subdivision in terms of trans- to interfere significantly with olfactory associative learning mitter expression (Schafer and Bicker, 1986; Schafer et al., 1988). Reduced silver staining (Heisenberg, 1980) and electron microscopy (Technau and Heisenberg, 1982) of *These authors contributed equally to this work. the Drosophila mushroom bodies reveal local differences Figure 1. Mushroom Body Organization (Top) Schematic representations of the Drosophila mushroom bodies as revealed by reduced silver staining. On the left is a frontal view of the right protocerebral hemisphere, showing dense clusters of Kenyon cell bodies (cb) above and behind the calyx (ca). The calyx is formed by Kenyon cell dendrites and afferents from the olfactory lobes. Beneath each calyx, Kenyon cell axons converge to form a stalk-like structure, the pedunculus (ped). This extends almost to the front of the brain, where it divides into a dorsally projecting a lobe and a b/r complex projecting toward the midline. On the right is a sagittal view of a mushroom body showing approximate planes of section in (a)-(f). Shown In fainter outline is another brain structure, the central complex. (a-f) g-Gal expression revealed by X-Gal staining in representative 12 trrn cryostat frontal sections through the head of P(GAL4] line 3OY. Bar, 10 urn. SubdivIsion of Drosophila Mushroom Bodies 47 in Kenyon fiber density and diameter. Can this be sufficient mushroom bodies of normal size and shape. Different pat- to account for the variety of proposed roles for mushroom terns must therefore represent GAL4 expression in differ- bodies in behavior, or does behavioral complexitY jmPlY ent, genetically specified subsets of Kenyon cells. a more complex neuronal organization? A powerful means of revealing neuronal complexity in Pattern Relationships at Different Levels Drosophila is the P[GAL4] enhancer-trap technique of Organization (Brand and Perrimon, 1993; Kaiser, 1993). An enhancer- Differences between patterns can be resolved at all levels trap element is a transposon containing a reporter gene. of mushroom body organization: cell body cortex, calyx, Insertions that occur in close proximity to a transcriptionat pedunculus, and lobes. The differences are primarily due enhancer cause the reporter to be expressed in a Pattern to longitudinal subdivisions of the volume encompassed that reflects the enhancer’s regulatory properties. Among by the mushroom body as a whole. This is most clearly a large collection of F[GAL4] lines, we found many with seen within the lobes and pedunculus. Longitudinal subdi- expression patterns corresponding to neuronaf trajecto- vision of the lobes is illustrated in Figures 2b-2d, in which ries, or architectures, familiar from classical neUrOanat- 3 lines exhibit three quite different staining patterns. Pro- omy. Here we describe lines in which reporter expression found longitudinal subdivisions of the pedunculus are evi- corresponds to the dendritic locations and axonal trajecto- dent in cross-sectional images obtained by confocal mi- ries of Kenyon cells, croscopy (Figures 3a-3d). They reveal groups of Kenyon cell axons to be organized concentrically, with one or more Results outer rings enclosing several discrete fiber bundles, Like the rings that surround them, each fiber bundle appears GAL4 Expression in Kenyon Cell Subsets to have a layered organization. We screened 1400 newly generated FIGAL lines for The general picture that emerges is of groups of Kenyon GALCdirected b-galactosidase (b-gal) expression in crYo- cell axons disposed at characteristic, often concentric, po- Stat frontal sections of the Drosophila head. p-Gal activity sitions within the pedunculus, beyond which they segre- iS readily detected as a blue stain produced by conversion gate to characteristic regions of the lobes. Consider first of the chromogenic substrate X-Gal or with anti-P-gal anti- projections to the y lobe. A spur-like structure is often ob- bodies Many lines had b-gal expression more or less re- served at the branch point of the pedunculus and the lobes stricted to specific anatomical domains of the brain, and (see Figures 2b and 2~). Staining of the spur provides in a number of lines the mushroom bodies Were clearly continuity between the outer ring(s) of the pedunculus and revealed. Figures la-if show a series of sections through the y lobe (see also Figure 4) an interpretation supported the brain of line 3OY, in which b-gal expression iS a good byconsiderationsof staining intensity. Thus, spur intensity match for the classical view of Drosophila
Details
-
File Typepdf
-
Upload Time-
-
Content LanguagesEnglish
-
Upload UserAnonymous/Not logged-in
-
File Pages10 Page
-
File Size-