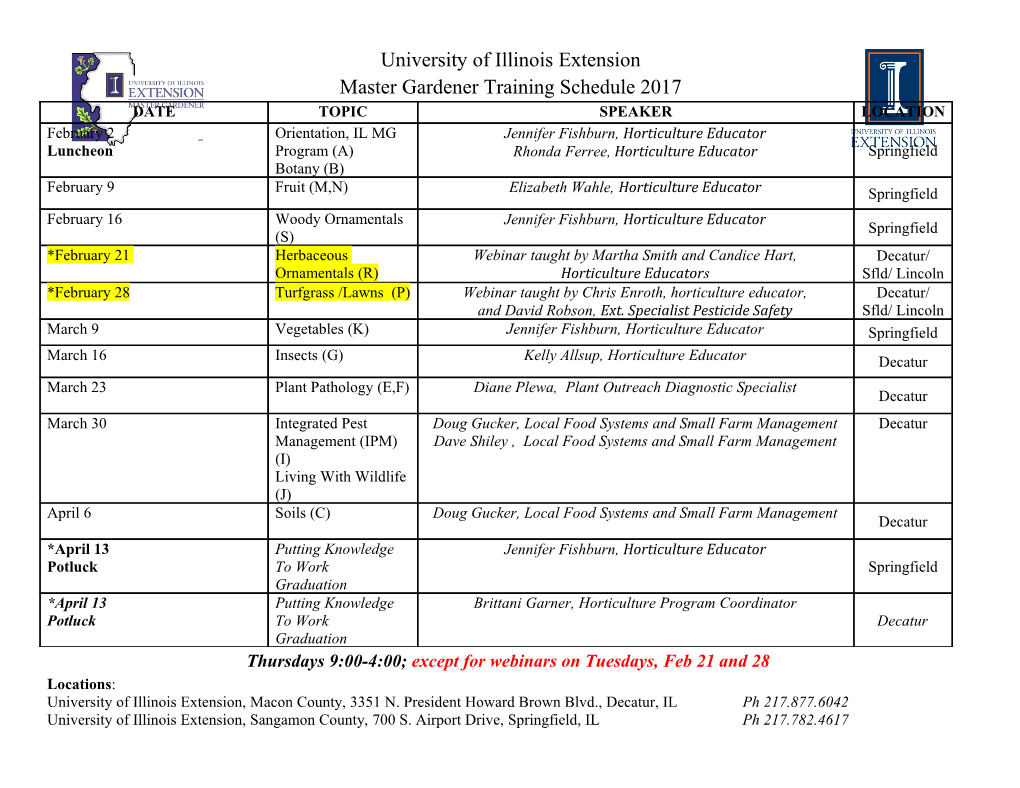
A PERFORMANCE BENCHMARK OF RECENT PERSONAL AIR VEHICLE CONCEPTS FOR URBAN AIR MOBILITY Michael Shamiyeh*, Raoul Rothfeld*, Mirko Hornung* *Bauhaus Luftfahrt e.V., Taufkirchen, Germany Keywords: eVTOL, Urban Air Mobility, Multicopter, Lift+Cruise, Performance Characteristics Abstract pulsion system [kW] P Hover power [kW] The prospect of a future Urban Air Mobility Hover PVTOL, Installed Installed power of the VTOL scenario brought up a vast number of according propulsion system [kW] vehicle programs. Due to a novel design space RoC Rate of climb [m/s] enabled by distributed electric propulsion, fun- RoD Rate of descent [m/s] damentally different vertical take-off and land- tLanding Hover time landing [s] ing aircraft concepts are developed. To further tTake-Off Hover time take-off [s] concretize the vision of an urban air transporta- VCruise Vehicle cruise speed [km/h] tion system, it is important to know the charac- VMaxRange Vehicle cruise speed for maxi- teristics of the various vehicle technologies and mum range [km/h] to understand the effects on an overall system. VTip, Max Maximum rotor tip speed [Ma] This technical paper evaluates two recent air- ζ C-Rate [1/h] craft concepts: an 18-rotor multicopter and a configuration with separate propulsion systems Abbreviations for vertical take-off and landing and fixed-wing cruise flight (lift+cruise). Starting from a base DEP Distributed Electric Propulsion configuration, several versions of the aircraft eVTOL Electric Vertical Take-off and Land- are derived to investigate sizing effects and ing (Vehicle) L+C Lift + Cruise trade-offs between VTOL and cruise efficiency. MC Multicopter Mission performance calculations for a defined MTOW Maximum Take-off Weight design mission and off-design missions are con- OWE Operating Weight Empty ducted. Finally, a demonstration scenario simu- PAV Personal Air Vehicle lation is presented, which will allow future in- PMAD (Electric) Power Management and vestigations regarding the transport perfor- Distribution Unit mance of a vehicle within a complete urban air UAM Urban Air Mobility transportation system. VTOL Vertical Take-off and Landing Nomenclature 1 Introduction Symbols The vision of Urban Air Mobility (UAM) is H Take-off/landing altitude [m] currently attracting a tremendous amount of 0 attention. As a possible solution for capacity HCruise Cruise altitude [m] L/D Lift-to-drag ratio [-] bottlenecks and/or attractive mobility alternative PCruise, MaxRange Cruise power at maximum range to ground transportation in urban areas, UAM is cruise speed [kW] a promising future market. Potential stakehold- PCruise, Installed Installed power of the cruise pro- ers from different industries initiate a series of 1 SHAMIYEH, ROTHFELD, HORNUNG versatile activities to make the vision become For the further development and specification of reality. possible UAM scenarios, it is essential to know Among these activities, the design and devel- fundamental characteristics such as performance opment of a suitable aircraft is of central im- potential, design limits or technology sensitivi- portance. ties of the vehicle morphologies. In addition, The new attractive technology options arising these aspects are the basis for an evaluation of with nearly scale-free [1] distributed electric the vehicle performance in an UAM overall propulsion (DEP) open up a large vehicle design system. It is important to understand how cer- space. On one hand, DEP reduces effort and tain aircraft characteristics such as hover effi- complexity of vertical take-off and landing ciency, cruise efficiency, cruise speed, range, (VTOL) aircraft to a manageable level. On the maintenance effort and vehicle complexity, other hand, new standards in safety and reliabil- physical dimensions or charging times affect a ity are predicted in connection therewith. Strong potential urban aerial transport system. improvements are also expected in terms of op- This technical paper addresses two recent vehi- erating costs [2] and aircraft noise emissions. cle concepts: An 18-rotor multicopter (MC) The technical possibilities face diverse and new configuration of the rotary-wing group based on requirements of UAM. Strict emission, noise the Volocopter 2X [6] and a lift+cruise (L+C) and safety regulations can lead to a new configuration published by Aurora Flight Sci- weighting of the classic aircraft design drivers. ences [7]. Lift+cruise aircraft have two separate Thus, for example, the aviation industry has to drive groups: a vertically oriented VTOL group deal with scenarios of mass production on the and a horizontally oriented cruise group provid- scale of automobile manufacturers. Despite the ing thrust for fixed-wing cruise. Referring to the publication of comprehensive UAM studies and wheel of V/STOL Aircraft and Propulsion Con- whitepapers, e.g. [3] [4], detailed boundary cepts [8], this vehicle class is in accordance to conditions for a target-oriented vehicle design the Lift+Cruise category there. are not yet in place. Even classical aircraft re- Both concepts are designed according to pub- quirements like range, cruise speed or seat ca- lished data using conceptual design methods pacity remain unclear. In the absence of precise and homogeneous underlying technology as- performance targets, a variety of fundamentally sumptions. Subsequently, several configurations different aircraft concepts referred to as electric of varying size are derived to illustrate sizing vertical take-off and landing vehicle (eVTOL), options and effects. In an off-design study the air taxi or personal air vehicle (PAV) were pre- transport energy efficiency of all configurations sented in the past years [5]. A two-step classifi- for different mission profiles is investigated. cation scheme depicted in Fig. 1 clusters the Finally, an initial demonstration case of a sys- variety of aircraft concepts according to lift pro- tem-wide transport analysis is presented. A fic- duction during cruise and the mechanism to tive UAM scenario for a small town in the Unit- enable VTOL. The aim is to obtain aircraft clus- ed States, Sioux Falls, is simulated. ters with comparable performance data and characteristics. 2 Methods, Modelling and Assumptions UAM VTOL Aircraft Vehicle design and mission performance are based on a combination of common conceptual aircraft design methods. In the following sub- Rotary-Wing Fixed-Wing Cruise Cruise section a compact overview of applied methods, implemented models and underlying assump- tions are presented. Tilt- Lift+Cruise Tailsitter Wing/Prop Fig. 1: Vehicle morphologies for VTOL aircraft [5]. 2 A PERFORMANCE BENCHMARK OF RECENT PERSONAL AIR VE- HICLE CONCEPTS FOR URBAN AIR MOBILITY 2.1 Aerodynamics The representation of these effects is essential, since especially during hover and vertical flight A blade element method with included tip loss high C-rates may occur. factor is used for VTOL rotors [9]. Cruise pro- pellers are designed with a method published by Adkins [10]. All rotors and propellers are calcu- 2.3 Mission Profile and Design Mission lated with underlying 2D aerodynamic polars of Mission performance analysis is based on a a CLARKY profile. simplified five-segment mission profile. Hover Lift, drag and moment coefficients of fixed segments with a defined duration, tTake-Off and wings are calculated with an adapted version of tLanding, represent take-off and landing. Climb the lifting line theory [11], allowing the integra- and descent are performed vertically from an tion of 2D profile polars. Parasitic drag is con- initial altitude, H0, to cruise altitude, HCruise, and sidered by equivalent flat plate areas [9]. vice versa with a rate of climb, RoC, and a rate of descent, RoD. The cruise segment consists of 2.2 Electric Power Train a horizontal flight at cruise altitude with con- The electric power train model consists of elec- stant cruise design speed of the respective vehi- tric motors, a central power management and cle. Fig. 3 shows the complete mission profile in distribution unit (PMAD) and battery packs. For overview. component weights and efficiencies, constant values are assumed, see Table 1. Table 1: Power train component characteristics. Component Specific Energy/ Efficiency Specific Power Battery 250 Wh/kg 97 % PMAD 7.0 kW/kg 95 % Fig. 3: Five-segment UAM mission profile. El. Motor 5.0 kW/kg 96 % Based on the mission profile, an UAM design In addition to the battery efficiency, which con- mission is defined with the parameters listed in siders battery management, for discharge char- Table 2. acteristics a runtime model of batteries is in- cluded [12]. Fig. 2 depicts the modelled dis- Table 2: Design mission parameters. charge characteristics of a single battery cell. Parameter Value Unit Discharging the battery with higher C-rates, ζ, tTake-Off 30 s reduces the extractable amount of energy of the tLanding 30 s battery significantly. H0 0 m HCruise 300 m RoC 2.5 m/s RoD 2.5 m/s Transition and acceleration/deceleration phases were neglected in the subsequent studies. Also neglected were climb and descent segments with forward speed. While the presented studies still provide meaningful results with the simpli- fied mission profile, the more detailed flight phases mentioned must be considered in future investigations. Fig. 2: Modelled discharge characteristics of a single battery cell for varying C-rate. 3 SHAMIYEH, ROTHFELD, HORNUNG 3 UAM Aircraft Platforms The original design (hereinafter referred to as MC Ultralight) has a maximum take-off weight For the studies presented below, both configura- (MTOW) of 450kg with a maximum payload of tions, the multicopter and the lift+cruise concept 160kg. The two-seater is certified as German are modelled according to published data. In ultralight in the class of light sport multicopter addition, derived versions of both vehicles are [6]. designed to evaluate sizing effects and scalabil- Based on the original configuration, two modi- ity. fied versions are derived: on one hand a slightly larger variant with 225kg payload (MC Light) 3.1 Multicopter Configurations and on the other hand a four-seater version fea- The considered multicopter design has 18 rotors turing 450kg maximum payload (MC Heavy).
Details
-
File Typepdf
-
Upload Time-
-
Content LanguagesEnglish
-
Upload UserAnonymous/Not logged-in
-
File Pages12 Page
-
File Size-