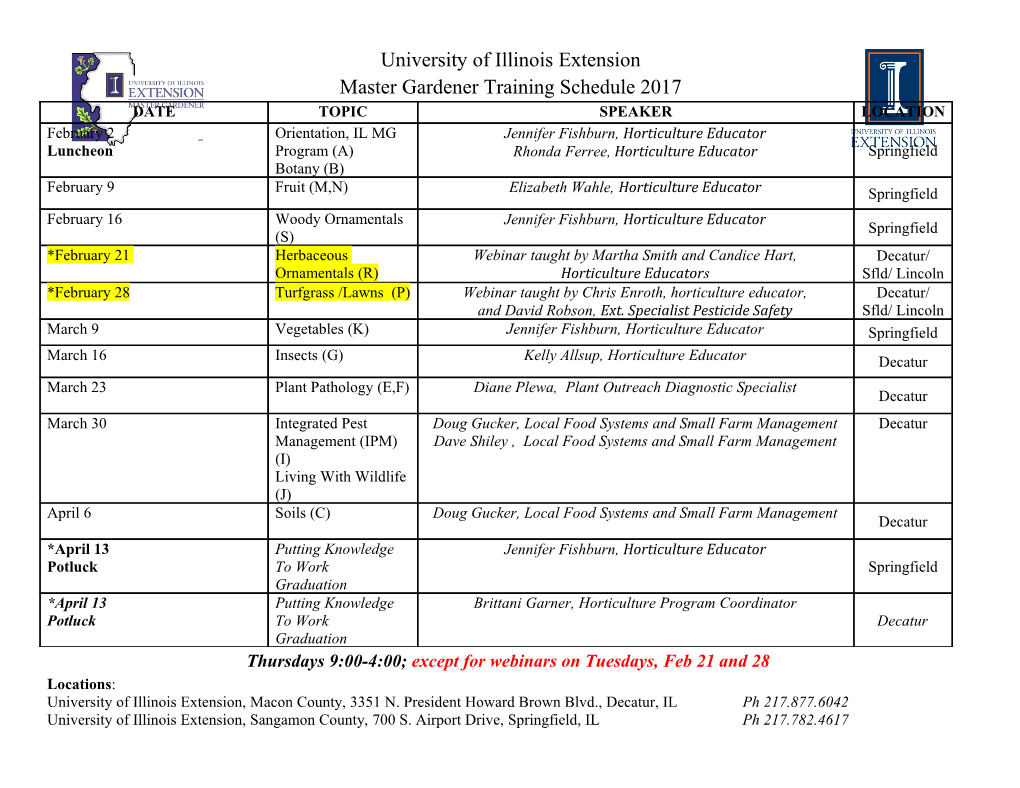
Pt-cisplatin 195m Ga-DOTATATE 68 and Else Aagje Aalbersberg Imaging biomarker development development biomarker Imaging and optimization of and optimization Imaging biomarker development and optimization of 195mPt-cisplatin and 68Ga-DOTATATE Else Aagje Aalbersberg Imaging biomarker development and optimization of 195mPt-cisplatin and 68Ga-DOTATATE Else Aagje Aalbersberg ISBN: 978-94-6323-813-7 © Else Aagje Aalbersberg Cover design and layout: © evelienjagtman.com Printed by: Gildeprint Imaging biomarker development and optimization of 195mPt-cisplatin and 68Ga-DOTATATE Ontwikkeling en optimalisatie van 195mPt-cisplatin en 68Ga-DOTATATE als biomarker (met een samenvatting in het Nederlands) Proefschrift ter verkrijging van de graad van doctor aan de Universiteit Utrecht op gezag van de rector magnificus, prof.dr. H.R.B.M. Kummeling, ingevolge het besluit van het college voor promoties in het openbaar te verdedigen op dinsdag 29 oktober 2019 des middags te 12.45 uur door Else Aagje Aalbersberg geboren op 16 oktober 1987 te Waalre Promotor: Prof. dr. G.D. Valk Copromotoren: Dr. M.P.M. Stokkel Dr. W.V. Vogel This research was performed at the department of Nuclear Medicine at the Antoni van Leeuwenhoek hospital, Amsterdam, the Netherlands. Printing of this thesis was financially supported by: 2Quart Medical BV Advanced Accelerator Applications ChipSoft Ipsen MILabs NKI-AVL NRG Pfizer CONTENTS CHAPTER 1. General introduction 9 CHAPTER 2. Preclinical imaging characteristics and quantification of Platinum- 19 195m SPECT Eur J Nucl Med Mol Imaging. 2017;44:1347-1354. CHAPTER 3. Toxicity, efficacy and tumour imaging of195m Pt-cisplatin in mice 37 Manuscript in preparation. CHAPTER 4. Pre-hydration in cisplatin-based CCRT: effects on tumour 53 concentrations and treatment outcome Radiother Oncol. 2019;134:30-36. CHAPTER 5. A practical guide to production and PET/CT imaging of 68Ga- 71 DOTATATE for neuroendocrine tumors in daily clinical practice J Vis Exp. 2019 Apr 17;(146). CHAPTER 6. Differences in uptake between68 Ga-DOTATATE and 68Ga-HA- 89 DOTATATE: a retrospective study in 342 patients Submitted and under review. CHAPTER 7. Influence of Lanreotide on Uptake of68 Ga-DOTATATE in Patients 107 with Neuroendocrine Tumours: a Prospective Intra-Patient Evaluation Eur J Nucl Med Mol Imaging. 2019;46:696-703. CHAPTER 8. Parameters to predict progression free and overall survival after 125 peptide receptor radionuclide therapy: a multivariate analysis in 782 patients J Nucl Med. 2019 [e-pub ahead of print]. CHAPTER 9. General discussion 145 ADDENDUM Nederlandse samenvatting 163 Dankwoord 171 Affiliations 177 Publications 183 Curriculum Vitae 187 Chapter 1 General introduction General introduction GENERAL INTRODUCTION 1 Cancer affects people worldwide, causing approximately 9.6 million deaths in 2018 globally, making it the second leading cause of death after cardiovascular disease.1 The socio-economic impact of cancer is enormous, and it was estimated that the worldwide cost of cancer was $1.16 trillion in 2010. Although cancer remains a deadly disease, the 10-year survival rate for all cancers has increased in the Netherlands from 32% in 1961-1970 to 54% in 2006-2010.2 This increase in survival is due to both early detection and improved treatment strategies, in which new anti-cancer drugs play a pivotal role. However, with the increasing number of drugs and drug-classes (chemotherapy, targeted therapy, immunotherapy, etc.) either approved or in development, other challenges present itself. The first challenge is selecting the most effective drug for a patient when many alternatives are available. Since ineffective treatment wastes valuable time and allows for disease progression, the most effective drug for an individual patient should be identified prior to the start of treatment based on patient- or tumor characteristics. This not only benefits individual patients, but also society as a whole since many modern therapies come at a high economic cost. The second challenge is choosing the drug with the least side-effects for a patient, without compromising treatment outcome. Side-effects can be severe, impact quality of life, and require dose adjustments, leading to sub-optimal treatment. The third challenge is performing response-assessment at the right time allowing for alterations of the treatment-regimen. Premature response-assessment can unjustly conclude that a drug is ineffective while late response-assessment risks disease progression under ineffective therapy. Nuclear medicine and quantitative imaging biomarkers could play a pivotal role in overcoming these challenges. Nuclear medicine Nuclear medicine employs radiopharmaceuticals for imaging of organ function or pathophysiology of disease. In contrast to anatomical imaging, nuclear molecular imaging provides information at the cellular or molecular level. These changes often present themselves on molecular imaging prior to anatomical changes which occur later in the course of a disease.3 Due to the nano- to picomolar sensitivity of nuclear medicine imaging modalities, tracer amounts of radiopharmaceuticals provide accurate information without interfering with the biological process that is being studied.4 Ideally, the half-life of the radioactive isotope that is used matches the duration of the biologically relevant distribution of the molecule being investigated. This ensures complete imaging of the desired physiological process without causing excess radiation dose.4 Furthermore, data can be acquired over time through dynamic or serial imaging. With the increasing number of molecules, linkers, and isotopes becoming available, and the advances in imaging technology, the field of nuclear medicine is diversifying and gaining importance in healthcare.3 11 Chapter 1 Imaging biomarkers When a nuclear medicine examination is used to diagnose or stage cancer or to predict or monitor treatment efficacy or toxicity, this is known as an imaging biomarker. A biomarker is defined as ‘a characteristic that is measured as an indicator of normal biological processes, or responses to an exposure or intervention, including therapeutic interventions.’5 Biomarkers in nuclear medicine often fall in the category of diagnostic biomarker, but are also used as monitoring biomarker, response biomarker, predictive biomarker, prognostic biomarker, or safety biomarker.5 Imaging biomarkers can provide non-invasive data for tumor detection, monitoring, and response assessment, and examine all pathological- and normal organ tissues in one non-invasive examination, even providing data on heterogeneity within a tissue.6 In order for an imaging biomarker to be applicable in healthcare, it should measure a relevant (biological) feature, be measured quantitatively or categorically, be both repeatable and reliable, and should be validated both technically and clinically.7 This requires standardized acquisition, reconstruction, and analysis protocols. For patient selection in clinical oncological care, predictive and prognostic nuclear imaging biomarkers are gaining importance. A classic example in nuclear medicine is radioactive iodine, in which scintigraphic uptake of iodine is demonstrated with a low-dose of 123I or 131I prior to high-dose therapy with 131I.8 In the past decade imaging of radiolabeled drugs, peptides, and antibodies has increased tremendously for treatment selection, with 68Ga-labeled peptides and 89Zr-labeled antibodies as frontrunners. Uptake of 68Ga-labeled peptides is a prerequisite for treatment with 177Lu-labeled peptides in both neuroendocrine tumors and prostate cancer, while 89Zr-labeled antibodies related to the immune system (immunoPET) are currently being explored for patient selection prior to immunotherapy. Imaging biomarkers not only provide essential information in routine clinical care, but can also be instrumental in clinical trials. In an era where oncological treatment is shifting from traditional chemotherapies to molecular therapies or personalized medicine, patient selection, or patient enrichment, in clinical trials has become increasingly important.9 Introducing (imaging) biomarkers as early as phase I trials could accelerate research and decrease the number of negative phase II and phase III trials and increase the a priori chance of success.4,7,9,10 Up-front selection of patients with (imaging) biomarkers could prevent drugs from being effective only in a sub-group of a larger clinical trial.11,12 When used appropriately, imaging biomarkers could enhance drug discovery, aid in smart clinical trial design to improve the clinical management of oncological patients. The concept of imaging biomarkers is identical for each different type of cancer or different therapy, but each requires its own radiolabeled molecule, imaging protocol, and (pre-)clinical testing and validation. In this thesis, the described aspects of biomarker imaging are explored for two different radiolabeled molecules.Part 1 of this thesis describes the first pre-clinical 12 General introduction steps for the introduction of 195mPt-cisplatin as an imaging biomarker for cisplatin treatment. Additionally, factors that influence the biodistribution of cisplatin are investigated.Part 2 1 focusses on 68Ga-somatostatin receptor imaging for neuroendocrine tumors, factors that influence the uptake of68 Ga-somatostatin receptor imaging, and the subsequent treatment with 177Lu-somatostatin analogues. Part 1: Cisplatin Cisplatin (cis-diamminedichloridoplatinum(II)) is a traditional chemotherapeutic drug that
Details
-
File Typepdf
-
Upload Time-
-
Content LanguagesEnglish
-
Upload UserAnonymous/Not logged-in
-
File Pages191 Page
-
File Size-