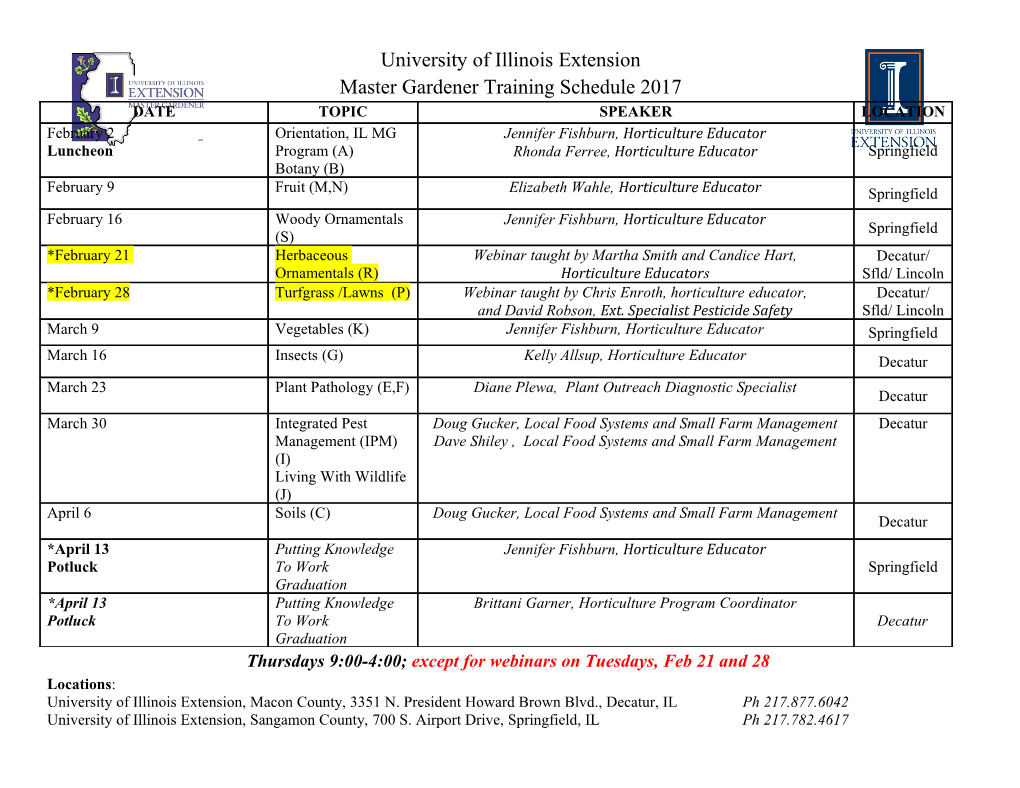
Neurometabolic Implications of Coenzyme Q10 Deficiency: Pathogenesis, Detection and Treatment Submitted By: Kate Duberley Department of Molecular Neuroscience UCL Institute of Neurology Queen Square, London Submitted July 2013 Funded by Ataxia UK Thesis submitted for the degree of Doctor of Philosophy, University College London (UCL) 1 I, Kate Duberley confirm that the work presented in this thesis is my own. Where information has been derived from other sources, I confirm that this has been indicated in the thesis. Signed………………………………………………….Date…………………… 2 Abstract Disorders of Coenzyme Q10 (CoQ10) biosynthesis represent the most treatable subgroup of mitochondrial diseases. Neurological involvement is frequently observed in CoQ10 deficiency, typically presenting as cerebellar ataxia and/or seizures. The aetiology of the neurological presentation of CoQ10 deficiency has yet to be fully elucidated and therefore in order to investigate these phenomena we have established a neuronal cell model of CoQ10 deficiency by treatment of the neuronal SH-SY5Y cell line with Para-AminoBenzoic Acid (PABA). This neuronal cell model provides insights into the effects of CoQ10 deficiency on neuronal mitochondrial function and oxidative stress. A marginal decrease in CoQ10 status (76% residual CoQ10) appears to be sufficient to impair Electron Transport Chain (ETC) function and increase mitochondrial oxidative stress, highlighting the vulnerability of neurons to a small deficit in CoQ10 status. In contrast to CoQ10 deficient fibroblasts, a CoQ10 deficiency (46% residual CoQ10) in neuronal cells appears to result in reversal of Complex V activity. This phenomenon has not been reported in previous studies of CoQ10 deficiency and may be a unique characteristic of neuronal cells. This neuronal cell model was subsequently utilised in the evaluation of candidate therapies for neurological conditions associated with CoQ10 deficiency. The efficacy of CoQ10 supplementation and methylene blue (MB) treatment were evaluated. CoQ10 supplementation proved effective at preventing mitochondrial oxidative stress and partially restoring neuronal mitochondrial function. However ETC complex activities were still compromised, suggesting an explanation for the refractory nature of neurological CoQ10 deficiency to treatment. Muscle is considered the “gold standard” for CoQ10 quantification; however neurological CoQ10 deficiency does not always present with a significant decrease in muscle CoQ10 status, despite a genetic diagnosis of CoQ10 deficiency. Cerebrospinal Fluid (CSF) CoQ10 quantification offers a more direct measurement of cerebellar CoQ10 levels. A tandem mass spectrometry (MS/MS) method capable of quantifying nanomolar (nM) levels of CoQ10 was therefore developed. 3 In conclusion this PhD thesis has been successful in expanding our understanding of the pathophysiology of neuronal CoQ10 deficiency and subsequently suggesting why neurological CoQ10 deficiency might be refractory to CoQ10 treatment. This thesis has also led to the development of a new technique for quantification of CSF CoQ10 concentration, opening up many possibilities for future studies and applications. This project is funded by Ataxia UK (www.ataxiauk.org.uk). 4 Table of Contents NEUROMETABOLIC IMPLICATIONS OF COENZYME Q10 DEFICIENCY: PATHOGENESIS, DETECTION AND TREATMENT 1 ABSTRACT 3 TABLE OF CONTENTS 5 TABLE OF FIGURES 11 LIST OF TABLES 15 LIST OF ABBREVIATIONS 16 ACKNOWLEDGEMENTS 19 CHAPTER 1 21 INTRODUCTION 21 1.1. COENZYME Q10 STRUCTURE 22 1.2. COENZYME Q10 FUNCTION 24 1.2.1. The Mitochondrial Electron Transport Chain (ETC) 24 1.2.2. Mitochondrial Oxidative Stress and the Antioxidant Role of Coenzyme Q10 36 1.2.3. The Plasma Membrane Redox System 41 1.2.4. Lysosomal Electron Transfer 41 1.2.5. The Mitochondrial Permeability Transition Pore (PTP) 42 1.2.6. Uncoupling Proteins (UCPs) 42 1.2.7. Cofactor in Pyrimidine Biosynthesis 43 1.2.8. Regulation of Cellular Membranes 43 1.3. COQ BIOSYNTHESIS 44 1.3.1. The Mevalonate Pathway 44 1.3.2. CoQ Biosynthesis 45 1.4. HUMAN COQ10 DEFICIENCY 49 5 1.4.1. COQ2 (encoding PHB-polyprenyl transferase) 50 1.4.2. PDSS1 and 2 (encoding transprenyl transferase) 51 1.4.3. ADCK3 (or CABC1) 51 1.4.4. COQ9 52 1.4.5. COQ6 52 1.4.6. COQ4 53 1.4.7. Secondary CoQ10 Deficiency 55 1.5. COQ10 SUPPLEMENTATION 58 1.5.1. Primary CoQ10 Deficiency 59 1.5.2. Secondary CoQ10 Deficiency 60 1.6. AIMS 61 CHAPTER 2 62 MATERIALS AND METHODS 62 2.1. MATERIALS 63 2.2. TISSUE CULTURE 64 2.2.1. Cell Line 64 2.2.2. Cell Passage 64 2.2.3. Cell Storage and Recovery 66 2.2.4. Treatment of SH-SY5Y Cells 66 2.2.5. SH-SY5Y Cell Harvesting for Biochemical Analysis 67 2.2.6. SH-SY5Y Cell Harvesting for Microscopy 67 2.3. TOTAL PROTEIN DETERMINATION 70 2.4. COENZYME Q10 QUANTIFICATION 70 2.4.1. Equipment 70 2.4.2. Procedure 70 2.4.3. Data Analysis 71 2.5. REDUCED GLUTATHIONE (GSH) QUANTIFICATION 74 6 2.5.1. Equipment 74 2.5.2. Procedure 74 2.5.3. Data Analysis 75 2.6. ENZYME ASSAYS 77 2.6.1. Complex I Assay (NADH:ubiquinone reductase; EC 1.6.5.3) 77 2.6.2. Complex II/III Assay (succinate cytochrome c reductase; EC 1.8.1.3) 78 2.6.3. Complex IV Assay (Cytochrome c oxidase; EC 1.9.3.1) 78 2.6.4. Citrate Synthase Assay (CS; EC 4.1.3.7) 79 2.6.5. Complex V (EC 3.6.3.14) in Gel Assay 82 2.6.6. Adenosine Triphospate (ATP) Assay 83 2.7. CONFOCAL MICROSCOPY 86 2.7.1. Preparation of Cells 86 2.7.2. Confocal microscopy method 86 2.7.3. Mitochondrial Membrane Potential 86 2.7.4. Mitochondrial Superoxide 88 2.7.5. Cytosolic Superoxide 88 2.7.6. Lipid Peroxidation 91 2.7.7. Mitochondrial DNA Quantification 91 2.8. STATISTICAL ANALYSIS 92 CHAPTER 3 95 NEURONAL CELL MODEL OF COENZYME Q10 DEFICIENCY 95 3.1. INTRODUCTION 96 3.2. AIMS 97 3.3. METHODS 98 3.3.1. Cell Culture 98 3.3.2. Quantification of CoQ10 Levels 98 7 3.3.3. Mitochondrial Bioenergetics Analysis 98 3.3.4. Blue Native in-Gel Complex V Assay 98 3.3.5. Oxidative Stress Assessment 98 3.3.6. Mitochondrial DNA Quantification 98 3.3.7. Mitochondrial Membrane Potential 98 3.3.8. Total Protein Determination 98 3.3.9. Statistical Analysis 98 3.4. RESULTS 99 3.4.1. Optimisation of PABA Treatment 99 3.4.2. Electron Transport Chain Activities 101 3.4.3. Cellular ATP Status 103 3.4.4. Blue Native Complex V Assay 103 3.4.5. Oxidative Stress Analysis 105 3.4.6. Mitochondrial DNA Quantification 106 3.4.7. Mitochondrial Membrane Potential 106 3.4.8. Co-incubation of PABA and CoQ10 109 3.5. DISCUSSION 112 3.6. CONCLUSION 116 CHAPTER 4 117 INVESTIGATION OF COENZYME Q10 AND METHYLENE BLUE TREATMENT ON THE NEURONAL CELL MODEL OF COENZYME Q10 DEFICIENCY 117 4.1. INTRODUCTION 118 4.2. AIMS 121 4.3. METHODS 121 4.3.1. Cell Culture 121 4.3.2. Quantification of CoQ10 Levels 121 4.3.3. Mitochondrial Bioenergetics Analysis 121 8 4.3.4. Oxidative Stress Assessment 121 4.3.5. Mitochondrial Membrane Potential 121 4.3.6. Total Protein Determination 122 4.3.7. Statistical Analysis 122 4.4. RESULTS 122 4.4.1. Treatment Optimisation 122 4.4.2. Coenzyme Q10 Treated Cells 124 4.4.3. Methylene Blue Treated Cells 128 4.5. DISCUSSION 131 4.6. CONCLUSION 134 CHAPTER 5 136 TANDEM MASS SPECTROMETRY METHOD FOR QUANTIFYING COENZYME Q10 IN CSF 136 5.1. BACKGROUND 137 5.2. AIMS 138 5.3. SYNTHESIS OF DEUTERATED COQ10 (D6-COQ10) 138 5.4. TANDEM MASS SPECTROMETRY METHOD DEVELOPMENT 139 5.4.1. Preparation of Standard Solutions 139 5.4.2. Subjects 140 5.4.3. Preparation of Patient Samples 140 5.4.4. Electrospray Ionisation-MS/MS (ESI-MS/MS) 144 5.4.5. High Performance Liquid Chromatography (HPLC) 147 5.5. RESULTS 154 5.5.1. Deuterated Internal Standard 154 5.5.2. Linearity, Precision, Recovery 155 5.5.3. Muscle Analysis 156 5.5.4. Fibroblast and CSF Analysis 157 9 5.6. DISCUSSION 159 5.7. CONCLUSION 162 CHAPTER 6 164 GENERAL DISCUSSION 164 6.1. FUTURE WORK 171 6.1.1. Neuronal Cell Model 171 6.1.2. Treatments 171 6.1.3. MS Method 172 6.2. REFERENCES 173 10 Table of Figures Figure 1.1 The Chemical Structure of Coenzyme Q10 (CoQ10)..........................22 Figure 1.2 The Mitochondrial Electron Transport Chain (ETC) + Complex V (ATP Synthase).................................................................................................26 Figure 1.3 Fatty acid oxidation pathway and the ETF/ETF-QO System............27 Figure 1.4 The Krebs cycle ...............................................................................29 Figure 1.5 Complex III/ Q cycle.........................................................................32 Figure 1.6 The Structure of Complex V (ATP Synthase) ..................................35 Figure 1.7 Redox States of CoQ10 Ubiquinone, Ubisemiquinone and Ubiquinol: the fully oxidized ubiquinone form (CoQ10); the radical semiquinone intermediate (CoQ10H·), and the fully reduced ubiquinol form (CoQ10H2). ........39 Figure 1.8 The Mevalonate Pathway-illustrating the synthesis of CoQ10 ..........46 Figure 1.9 CoQ Biosynthesis ............................................................................54 Figure 2.1 Haemocytometer .............................................................................65 Figure 2.2 SH-SY5Y Neuroblastoma Cells stained with Bodipy 581/591, a Lipid Peroxidation Marker..........................................................................................68 Figure 2.3 A typical Bovine Serum Albumin (BSA) standard line......................69 Figure 2.4 A Typical CoQ10 Chromatogram for a SH-SY5Y Cell Sample..........72 Figure 2.5 Concentration Curve for CoQ10 (HPLC-UV Detection Method)........72 Figure 2.6 Schematic Diagram of a High Performance Liquid Chromatography (HPLC) System.................................................................................................73 Figure 2.7 A Typical Reduced glutathione (GSH) Chromatogram for a SH-SY5Y Cell Sample.......................................................................................................75 Figure 2.8 Reduced Glutathione (GSH) A) Voltamagram- utilised to find the optimum electrochemical detector (EC) electrode voltage.
Details
-
File Typepdf
-
Upload Time-
-
Content LanguagesEnglish
-
Upload UserAnonymous/Not logged-in
-
File Pages204 Page
-
File Size-