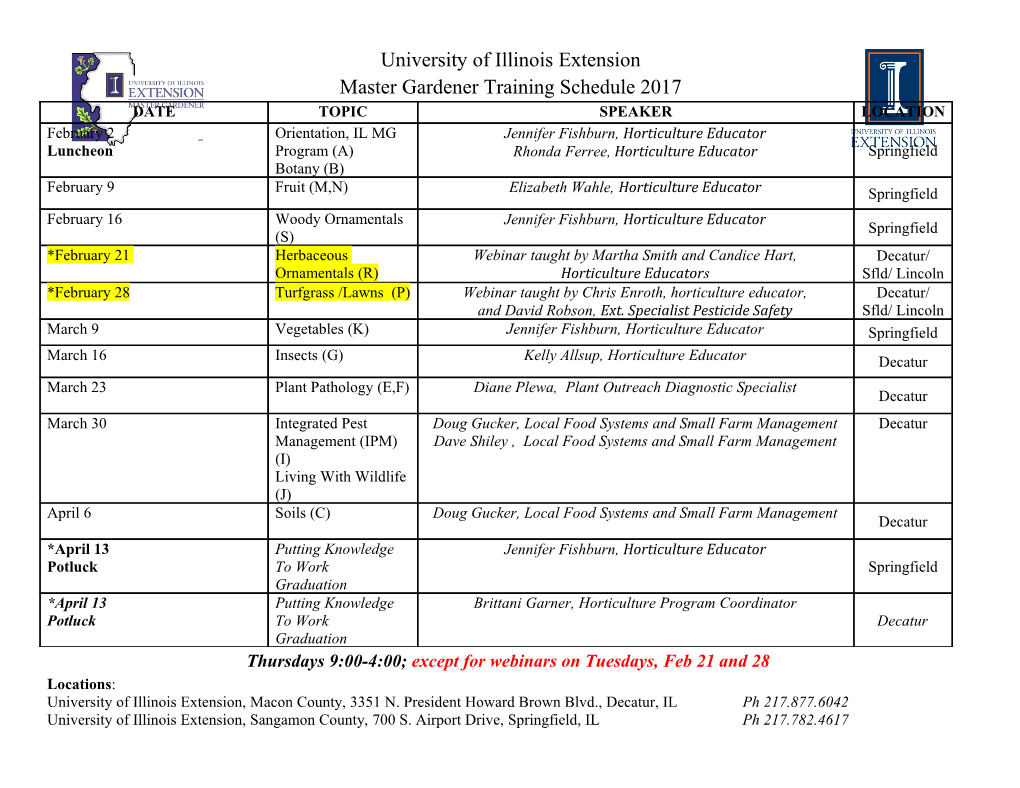
Today, dark matter Tomorrow the baryons Some of the constrained regions in the dark matter parameter space Mass 10−22 ev 1033 kg cdm Self Interaction collisionless collisional cdm Interaction with Photons CMB/Small None scale structure Interaction with Baryons terminal None velocity of cdm HVC’s Annihilation Cross Section 0 < core radius cdm Temperature Cold Hot cdm 1 kev Decay > T (Hubble) < T (Hubble) Using tidal tails to constrain dark matter halos Using tidal tails to constrain the nature of dark matter We can use Pal 5 to rule out massive compact objects = 10^4Mo black holes. The GC would very quickly be destroyed, but since we don’t know the initial GC mass function we can use the existence of the old narrow stream – this feature would be heated into a much larger feature. Some of the constrained regions in the dark matter parameter space Mass 10−22 ev 1033 kg cdm Self Interaction collisionless collisional cdm Interaction with Photons CMB/Small None scale structure Interaction with Baryons terminal None velocity of cdm HVC’s, moon Annihilation Cross Section 0 < core radius cdm Temperature Cold Hot cdm 1 kev Decay > T (Hubble) < T (Hubble) Celine Boehm etal (2002) astroph0112522 The Hubble sequence: from disks to spheroidals cD and lots of dE’s LSB MW dSph Rotation curves provide the oldest and most direct test for the dynamical mass distribution around galaxies. 22 GMm// r= mvc r vGMrc = / 32− ρρ(∝rMrrvconstrr)()/, c = →() ∝ vrc ∝→ρ( rconst ) = Trivial dynamics tells us a lot about the amount and distribution of dark matter within spiral galaxies. Unfortunately, it doesFraternali not tell etal us (2002)about the extent or shape of the dark matter distribution around galaxies. Dark matter in spiral galaxies: The HI rotation curve of NGC 2403 can be fit in several different ways, demonstrating the degeneracy in the halo/disk contributions. Bolatto, Blitz etal (2001) Confrontation of rotation curves with predictions from numerical simulations DeBlok, McGaugh, Rubin & Bosma (2002) Swaters, Madore, van den Bosch & Balcells (2002) MassMass profilesprofiles inin clustercluster corescores Presence of radial and tangential arcs of known z strongly constrains mass on 20-50 kpc scales MS2137-23 (z=0.313) Sand, Treu & Ellis (2002) Best-fitting density profile for MS2137-23 Powerful method: combines lensing (DM) and dynamics (light) on optimal scales for a cluster core LikelihoodLikelihood FitFit forfor DMDM profileprofile inin MS2137MS2137--2323 Treating cluster as Lensing only two-component ↑ system (luminous + DM), constrain β β over r <75 kpc in terms of M/L of stellar component: flatter slope than Lensing + dynamics indicated in CDM simulations NB: Baryonic collapse should steepen DM profile from original value M/LV → Constraints on mass profiles: hydrodynamics of X-ray gas Lewis etal (2002) – A2029 Inner slope -1.1 ignoring adiabatic contraction, can’t probe to the smallest scales. McKay etal (2001) Sloan Survey 31,000 galaxies • Mass-to-light ratio constant • Halos extend to 200kpc Multiply lensed quasars show evidence for substructure in the halo If the lensing object is smooth, then there exists a simple relation between the flux ratios: A/B=C/D=1 for a doublet, A/(B+C)=1 for a triplet Observed values of the flux ratios are in the range 0.6-0.8!!! McKay etal (2001) Sloan Survey 31,000 galaxies • Mass-to-light ratio constant • Halos extend to 200kpc A nearby well studied cluster of galaxies - the Local Group (Eva Grebel) Leo I 6 dSph 10 stars Omega-Cen globular cluster Globular clusters contain the same number of stars as a dSph galaxy but are 100 times smaller and do not show any evidence for dark matter. 2 kpc Clusters: M_total = 10^15Mo, R=1000kpc, σ=1000km/s Mdark : Mgas : Mstar = 100:10:1 Galaxies: M_total = 10^12Mo, R=100kpc, σ =100km/s Mdark : Mgas : Mstar = 100:1:1 Mass Dwarf spheroidals: M_total = 10^8Mo, R=1kpc, σ =10km/s Mdark : Mgas : Mstar = 100:0:1 Globular clusters: Dark matter M_total = 10^6Mo, R=10pc, σ =10km/s in virialised structures Mdark : Mgas : Mstar = 0:0:1 What we know about dark matter ...not much….. And that’s after about 10,000 papers…. Its dark and non-baryonic Dominates the kinematics of all galactic systems Traces the light (little bias, no dark galaxies/clusters) Masses and sizes of halos consistent with spherical collapse Density profiles inconsistent with spherical collapse Ωtotal : ΩΛ : Ωdark : Ωbaryon = 1 : 0.7 : 0.25 : 0.03 What we don’t know about dark matter ...plenty Are dark matter halos spherical or triaxial? Is the dark matter smooth or clumpy? Is their a maximum phase space density? How much mass is attached to galaxies in clusters? Is the dark matter clustered on scales < dSph? What is the contribution of dark baryonic material? Could the dark matter be cold, warm, fluid....? Fluid Dark Matter Spherical halos: may be good for streams No -ve J_z : good for disk formation Central core: interesting Few satellites: interesting A better concordance model?? The formation and future of the Local Group of galaxies z=-1 This is a tiny dark matter halo Dracothat would surround the Galactic dSph galaxies We can zoom into a patch of the Universe at very high spatial and mass resolution. 3kpc 10 solar mass per particle 10 parsecs force resolution 10^7Mo Moore etal (2001) A galaxy or cluster? Ghigna etal (1998) Lessons from pure dark matter simulations Substructure I Substructure II The abundance of satellite halos in CDM clusters and galaxies compared to data Vc/V/Vglobal The red regions marked at z=10 are the ≈100 halos that have already collapsed and could form stars. These regions are then traced to the final time where most of these halos have merged into the galaxy with about 10 satellites surviving in the final halo. z=10 z=0 Most of the satellites remain dark since they could not cool gas after re-ionisation. Problem: where are the smaller satellites with velocity dispersions less than 10 km/s? Does this work with early re-ionisation? Some of the constrained regions in the dark matter parameter space Mass 10−22 ev 1033 kg cdm Self Interaction collisionless collisional cdm Interaction with Photons CMB/Small None scale structure Interaction with Baryons terminal None velocity of cdm HVC’s Annihilation Cross Section 0 < core radius cdm Temperature Cold Hot cdm 1 kev Decay > T (Hubble) < T (Hubble) Evolution of a dark fluid halo Important results from hierarchical clustering simulations •Self similar structure •Overmerging resolved •Halos are triaxial, small J •Cuspy cores ρ(r)∝r^(-1.3) •Mergers are very rare in virialised halos •Tidal stripping works as expected A zoom into a rotating dark matter halo @Ben Moore (1999) (5-10% of mass attached to substructure) •Steep mass spectrum of subhalos n(m)∝m^-2 •Halos within halos are on isotropic orbits (half of halos are on orbits more radial than 5:1) nfw Dubinski & Carlberg (1991) Ghigna etal (2000) Disks cD Six months of supercomputer time in 1999 Confrontation of central density profiles with CDM numerical simulations Swaters, Madore, van den Bosch & Balcells (2002) Sand, Treu & Ellis (2003) Data from high resolution CDM halos measured at 1% R_200 rotation curves and clusters With reasonable resolution. Data right, CDM wrong Data interpretation wrong Explore new dark matter candidates Formation before re-ionisation WDM dSph masses much larger FDM Violent baryonic feedback SIDM Bar-halo response MDM Rotation curves hard to interpret SFDM Cluster central dynamics? ADM MOND What have we learnt from a comparison of observations with simulations? Its not easy comparing dark matter distributions with observations of the light Galaxies are better fitted with constant density cores, but cusps can not be ruled out. Observations suffer from non-circular motions and resolution effects. Clusters are better fit with central cores but cusps also consistent with the observations. Comparison is difficult because the predicted potential is non- spherical. Bottom line, CDM almost ok, but also nearly every other candidate particle is consistent with the observational data as well. Cold collapse and the core catastrophe The density profiles of cold, warm and hot dark matter halos of the same mass and formation history Changing the power spectrum can easily change the amount of substructure but not the final density profile. c.f. Huss et.al. (1999), Moore et.al. (1999) It’s a hard numerical problem… Klypin, Kravtsov, Primack (1999) – found constant density cores r −1 Density profiles and derivatives – ART, GRAPE, GADGET, PKDGRAV, TPM Diemand etal (2004) Convergence tests for the density profiles Fits to functions proposed in the literature Two parameter functions fits the data very well Generalised cusp Caution: Hierarchical structure formation implies that the first halos to collapse contain just a few particles. This is not a smooth representation of the CDM particle fluid and leads to relaxation. The green disk of massless points should stay infinitely thin given correct modelling of the system. N=10,000 equilibrium Galactic halo model Juerg Diemand etal (2003) Initial cuspy model After 3 mean relaxation times Also quantified relaxation as a function of radius, softening and degree of virialisation. Tested a local estimator based on the local phase space density so that we can track relaxation within a hierarchical simulation. Diemand etal, 2003 Relaxation during the Relaxation hierarchical clustering in an dominates the numerical isolated effects. 10,000 particle Increasing the number of CDM halo Relaxation from the full particles does not linearly hierarchical formation reduce relaxation as expected. Even with >10^5 particles the average degree of relaxation is greater than unity throughout the halo. 10^5 particle Relaxation tends to make islolated halo the problem worse for CDM since equipartition tends to flatten cusps.
Details
-
File Typepdf
-
Upload Time-
-
Content LanguagesEnglish
-
Upload UserAnonymous/Not logged-in
-
File Pages79 Page
-
File Size-