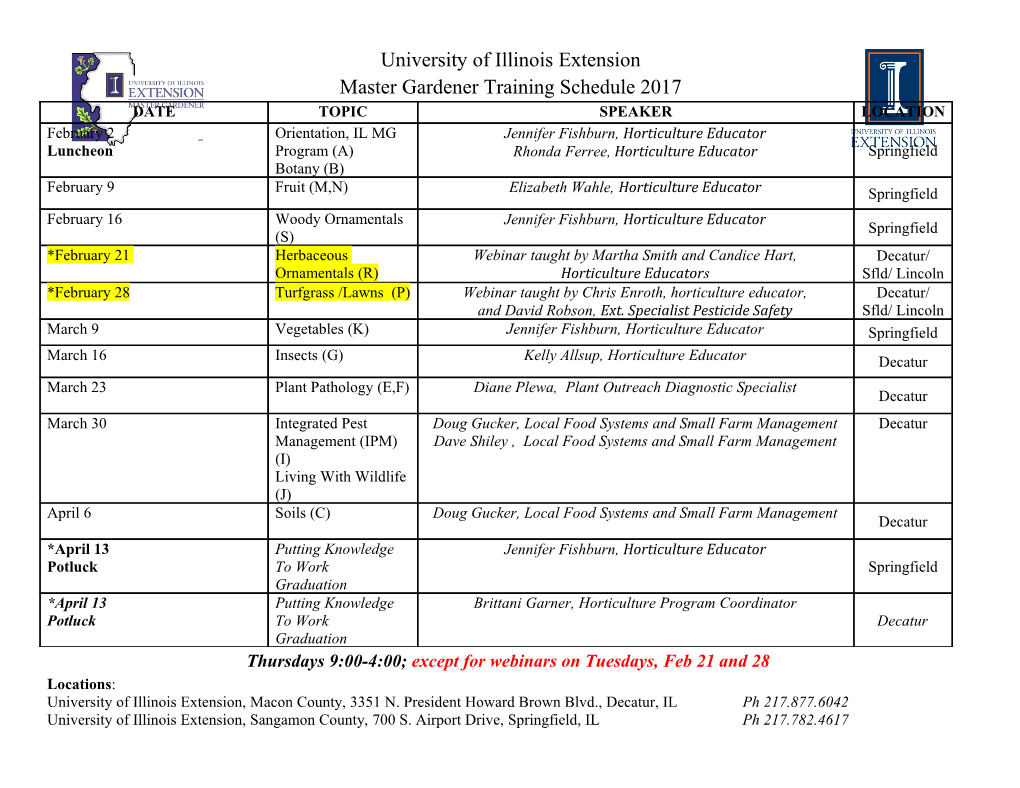
The Evolution of Cars; The Most Efficient Model Abstract: This project was designed to examine the effect of aerodynamics on a car’s fuel efficiency. The hypothesis was that car’s designed for speed, and acceleration would have a more refined aerodynamic design. After researching, it was found that the effect of refined aerodynamics are negligible at low speeds since the effect of the tires’ friction against the ground is much greater than the effect of wind resistance. At higher speeds it is the reverse. Refined aerodynamics contributed to improving the fuel economy by up to 20%. In order to test the hypothesis, it was determined that the experiment required wind resistance equivalent to highway speeds (62 mph) in to solely isolate the effect of the car’s shape in determining the drag force. The experiment consisted of two model cars under the pressure of simulated highway speeds via a leafblower. A force meter was attached to the models, measuring the drag force of the model. Two cars, a Volkswagen New Beetle and a 1957 Corvette C1 were used, two very different vehicle designs. Although the 1957 Corvette was designed for speed and acceleration, the more smoothly shaped Volkswagen New Beetle was more efficient. The gradual slope of the hood allowed the Beetle to achieve a drag force of 25 N, while the Corvette C1 has a drag force of 50 N. The lower drag force on the Beetle suggests that its design is more aerodynamic than the C1. The sloped angle of the hood on the Beetle can be adapted to the designs of modern SUVs and minivans to increase the fuel efficiency of all vehicles, which preserves the environment by reducing CO2 emissions. Question: What car design is the most efficient by aerodynamic design? Hypothesis: The most fuel efficient car design is slim and light, similar to sports cars. SUVs and Minivans will be much less aerodynamic, causing less fuel efficiency from the design itself. Background Research/Problem: Aerodynamics are a vital part in making a car more efficient. Automotive engineers work optimize the shape of the car in order to make it the least resistant to wind when driving. Optimizing automotive aerodynamics reduce in fuel consumption and improvement of driver control. A car’s ability to resist wind is often measured in advertised by its drag coefficient. The drag coefficient is determined experimentally and is dependant on the shape and friction of the surface of the car. This wind resistance is measured in drag. Drag is calculated by finding half the product of the density of the substance it is moving in, speed of the object relative to the substance, the cross sectional area, and the drag coefficient. This drag can be minimized by reducing the cross sectional area of the car. It can also be minimized by streamlining the shape of the car, creating the least amount of sharp angles or jagged edges that can be obstacles to the flowing wind. Finally, by lowering the body of the car to the ground, it can minimize the amount of lift force on the car when driven at high speeds. At higher speeds, this friction of the surface will have less of an effect on the drag of the car. This also pertains to the tire’s friction on the road. At higher speeds the resistance of the tires on the road becomes negligible. This is due to a high Reynolds number. Reynold’s number is used in fluid dynamics and measures the ratio between the inertia force of the object and the viscous force of the substance it is moving in. It’s purpose is to evaluate an object’s resistance to viscous forces of a substance. In the case of automotive aerodynamics, the viscous force would be the viscosity of the air. A high Reynold’s number means that the object has greater inertia. The inertia of the car, depends on its kinetic energy therefore an high speed would make the viscosity less significant in the effect on the object. This explains why at a higher velocity, the friction on the surface of the road, and the exterior of the car would be insignificant when calculating the drag at higher speeds. Experimentation and analysis will focus on higher speeds in order to eliminate factors that can not be controlled in the experiment such as the road friction and the surface of the models themselves. It focuses the experiment on the effect the shape of the car has on the aerodynamics. At highway speeds, only 30% of gas consumed is actually used to turn the wheels of the car. Of this 30%, 20% is used to overcome wind resistance. By engineering a car so that it has less drag, the fuel economy of a car can be improved. Just by reducing a car's coefficient of friction of by 0.01, the car can travel almost a quarter of a mile farther per gallon of gas. This is not the only factor in improving a car’s fuel economy, however, aerodynamics are vital to ensuring the optimal amount efficiency when traveling at high speeds. Materials: Computer with LoggerPro installed LoggerPro force meter String Leaf blower, Model 57’ Corvette C1 (1:32 of the size) Model Volkswagen Beetle (1:32 of the size) Anemometer Procedure: 1. Attach LoggerPro force meter to a computer 2. Determine wind speed by blowing the leaf blower on the anemometer 3. Tie string to the receiving end of the force meter 4. Tie opposite end of the string to a car model, so that the car is facing toward the force meter 5. Begin recording data by pressing “Start” on LoggerPro 6. Use leaf blower to provide a constant air force to the rear of the model car, blowing away from the force meter, record results 7. Repeat steps 4 - 6 with the Volkswagen Beetle Data/Results: Volkswagen New Beetle Drag Force 1957 Corvette C1 Drag Force Data Analysis: As a result, it is obvious that the Volkswagen New Beetle is more aerodynamic, despite its seemingly spherical shape when compared to that of the 1957 Corvette C1. When the leaf blower blew a highway speed resistance of 62 miles per hour, the drag force on the Corvette was about 0.35 Newtons more than that of the Beetle. After continuous trials we found that this trend stayed the same. The greater fluctuation in the graph if the wind resistance on the Corvette is due to the fact that the air has to travel a more indirect path, leading to less consistency in the reading. Conclusions: The force of the 57’ Corvette was higher than that of the Beetle because the cabin of the sports car rises from the hood at a sharp angle allowing the air to flow in a less direct or streamlined path resulting in more resistance. The angle of the cabin, smoothly rises from the Beetle, similar to vehicles such as sedans.The Beetle is also lower to the ground resulting in less lift forces when traveling at higher speeds. These results are consistent with the actual values of the drag force and frontal area for each of these cars. A Volkswagen has: a drag coefficient of 0.38 a frontal area of 1,581.0 inches squared a highway fuel efficiency of 28 miles per gallon While a 57’ Corvette C1 has: a drag coefficient of 0.48 a frontal area of 3,712.8 inches squared a highway fuel efficiency of 16 miles per gallon The Beetle’s drag coefficient alone accounts for 2.5 miles per gallon of this change seeing as it is 0.1 less than that of the Corvette. This is because for every 0.01 decrease in drag coefficient there is also increase in the fuel efficiency of an extra quarter mile per gallon. Also, the 57’ Corvette has more than twice the frontal area than that of the Beetle. This combined with the increase in weight and engine type result in a 14 mile per gallon fuel difference at highway speeds. Although not the entire 14 mile per hour difference is accounted for by the decrease in drag, it is important in optimizing the efficiency of other technologies also designed to improve the fuel economy such as the engine and wight. We concluded that this means that the Beetle is able to better optimize the efficiency of its engine. At highway speeds, 20%-30% of gas used is to overcome wind resistance. Therefore, because the aerodynamics are more refined on the Volkswagen, its fuel usage used to overcome wind resistance is on the lower end of the spectrum. The Volkswagen New Beetle is the more efficient car because of its more streamlined body, allowing for wind to travel in a more direct path, thus creating less of a drag force. Applications/Research: This experiment deals with the issue of rising gas prices and depleting fossil fuels. By knowing the most aerodynamic and fuel efficient car design, not only do consumers pay less at the pump, but also fossil fuels can be further conserved for future generations. Further research of this issue would include combining the design of the car with the car’s components. Similar experiments can be conducted to test ideal tire patterns to best grip the road surface, ideal engine types, etc. Only by combining all of the ideal aspects of a car can the ideal car design be achieved. From an experimental standpoint, further research could be done by testing more than just the two car shapes tested. A more streamlined body could be tested as well as an object with a higher drag coefficient.
Details
-
File Typepdf
-
Upload Time-
-
Content LanguagesEnglish
-
Upload UserAnonymous/Not logged-in
-
File Pages7 Page
-
File Size-