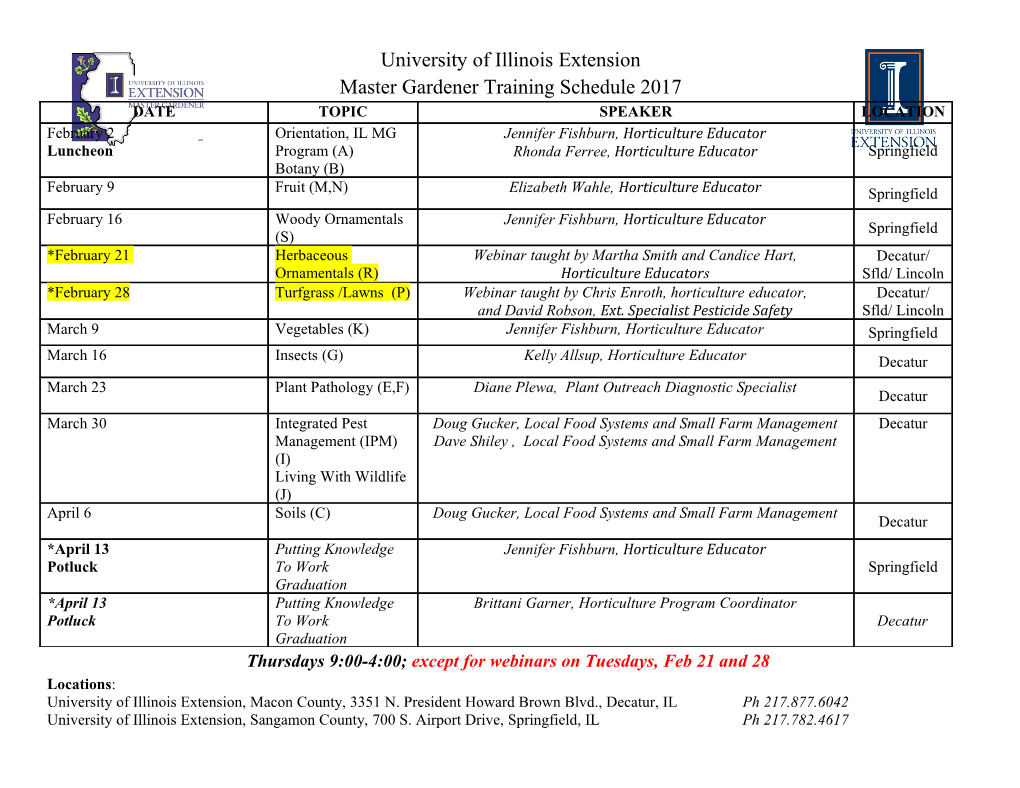
International Journal of Molecular Sciences Review Self-Amplifying RNA Viruses as RNA Vaccines Kenneth Lundstrom PanTherapeutics, CH1095 Lutry, Switzerland; [email protected] Received: 30 June 2020; Accepted: 16 July 2020; Published: 20 July 2020 Abstract: Single-stranded RNA viruses such as alphaviruses, flaviviruses, measles viruses and rhabdoviruses are characterized by their capacity of highly efficient self-amplification of RNA in host cells, which make them attractive vehicles for vaccine development. Particularly, alphaviruses and flaviviruses can be administered as recombinant particles, layered DNA/RNA plasmid vectors carrying the RNA replicon and even RNA replicon molecules. Self-amplifying RNA viral vectors have been used for high level expression of viral and tumor antigens, which in immunization studies have elicited strong cellular and humoral immune responses in animal models. Vaccination has provided protection against challenges with lethal doses of viral pathogens and tumor cells. Moreover, clinical trials have demonstrated safe application of RNA viral vectors and even promising results in rhabdovirus-based phase III trials on an Ebola virus vaccine. Preclinical and clinical applications of self-amplifying RNA viral vectors have proven efficient for vaccine development and due to the presence of RNA replicons, amplification of RNA in host cells will generate superior immune responses with significantly reduced amounts of RNA delivered. The need for novel and efficient vaccines has become even more evident due to the global COVID-19 pandemic, which has further highlighted the urgency in challenging emerging diseases. Keywords: RNA vectors; RNA replicons; RNA vaccines; immune responses; protection against pathogens; protection against tumor challenges 1. Introduction RNA-based vaccines have become potentially promising as alternative approaches to conventional vaccine development [1]. In this review, emphasis has been placed on tumor vaccines, but description of vaccines against pathogenic viruses has also been included as more progress has been made in this area, which can serve as encouragement for treatment and prevention of various types of cancer. Particularly, the utilization of mRNA due to its high potency, rapid production and development and safe administration has received plenty of attention. However, progress has been hampered by issues related to instability and low efficiency of in vivo delivery. Recent improvements in the field addressing optimization of mRNA translation and stability, modulation of immunogenicity and progress in mRNA vaccine delivery have contributed to vaccine platforms against infectious diseases and different types of cancers, showing encouraging results in both animal models and clinical trials. In this context, an mRNA vaccine targeting the rabies glycoprotein provided protection against lethal challenges with rabies virus in immunized mice and pigs [2]. In another study, mice and primates immunized with 30 µg and 50 µg of liposome-encapsulated nanoparticles containing mRNA for Zika virus (ZIKV) membrane and envelope glycoproteins, respectively, were protected against ZIKV challenges [3]. Moreover, lipid nanoparticle-encapsulated mRNA expressing influenza A virus hemagglutinin (HA) protected mice from lethal influenza virus challenges and reduced viral titers in ferret lungs [4]. Initial phase I clinical trial results demonstrated robust prophylactic immunity in humans with mild or moderate adverse events only. In another phase I clinical trial, mRNA encoding rabies glycoprotein was administered to healthy volunteers showing safety and a reasonable Int. J. Mol. Sci. 2020, 21, 5130; doi:10.3390/ijms21145130 www.mdpi.com/journal/ijms Int. J. Mol. Sci. 2020, 21, 5130 2 of 29 tolerability profile [5]. Functional antibodies against the viral antigen could be induced and boosted when administered with a needle-free device but not when a needle-syringe was used. In the context of mRNA-based vaccines, self-replicating RNA viruses present an interesting and attractive alternative as described below [6]. The common feature of self-amplifying RNA viruses is their single-stranded RNA (ssRNA) genome embedded in a capsid and envelope protein structure [6]. In the case of alphaviruses and flaviviruses, the ssRNA genome is of positive polarity in contrast to measles viruses (MVs) and rhabdoviruses, which carry a negative sense ssRNA genome. In any case, the RNA genome of self-amplifying RNA viruses can act directly in the cytoplasm without any need of nucleic acid delivery to the nucleus. In the case of positive polarity, the translation can be initiated directly from the incoming ssRNA genome [7], whereas negative sense RNA molecules require the generation of a positive strand RNA template [8]. All self-amplifying RNA viruses initially express their nonstructural genes resulting in the formation of the RNA replication complex (RNA replicon), responsible for extreme RNA replication in infected host cells [7]. It has been estimated that 200,000 copies of RNA are made from a single RNA molecule, providing together with strong subgenomic promoters the basis for extremely high expression levels of viral proteins. This feature has been taken advantage of in expression vectors engineered from self-amplifying RNA viruses, which have been applied for mammalian and non-mammalian cell lines, primary cells and in vivo [9]. Moreover, self-amplifying RNA virus vectors have been used for vaccine development, targeting both infectious diseases and different types of cancers. In this review, the basic function of self-replicating RNA virus vectors is described, and various preclinical and clinical applications are presented. 2. Self-Amplifying RNA Virus-Based Expression Although efficient expression systems have been developed for alphaviruses, flaviviruses, MVs and rhabdoviruses, the differences in the polarity of the ssRNA genome have required alternative engineering. In the case of alphaviruses and flaviviruses, the procedure is straightforward, whereas MVs and rhabdoviruses require the utilization of packaging cell lines and reverse genetics. For simplicity, the focus is here on the illustration of the Semliki Forest virus (SFV)-based expression system, one of the most commonly applied alphavirus expression systems (Figure1). Alphaviruses belong to the family of Togaviruses [7]. Particularly, SFV [10], Sindbis virus (SIN) [11] and Venezuelan equine encephalitis virus (VEE) [12] have been used for the engineering of expression vector systems. In this context, engineering of an expression vector carrying the SFV nonstructural protein genes (nsP1-4), where the gene of interest can be inserted downstream of the strong 26S subgenomic promoter allows in vitro transcribed RNA to be directly translated in cell lines and in vivo (Figure1). Alternatively, replacement of the SP6 or T7 RNA polymerase promoter upstream of the nsP1-4 region with a cytomegalovirus (CMV) promoter permits direct use of plasmid DNA in vitro or in vivo [13]. Another possibility is to co-transfect in vitro transcribed RNA from the expression vector and a helper vector expressing the SFV structural protein genes into mammalian host cells for production of replication deficient SFV particles. The reason for replication deficiency relates to the presence of the packaging signal in the nsP2 region of the expression vector, which prevents the packaging of the RNA from the helper vector [14]. Additionally, to reduce the formation of any replication proficient SFV particles, the second generation pSFV-Helper 2 vector was engineered [15]. Furthermore, for total elimination of RNA recombination and generation of replication proficient SFV particles a two-helper system with the capsid and spike protein genes on separated vectors was applied [16]. Finally, a full-length SFV genome with a second 26S subgenomic promoter and gene of interest introduced either downstream of the nsP genes or the structural genes can be applied, keeping in mind that replication proficient particles will be generated, obviously enhancing biosafety risks. In any case, the options and flexibility are excellent as alphavirus vectors can be used for vaccine development in the form of naked RNA replicons, DNA plasmid vectors and recombinant viral particles as described in more detail in the next sections. Int. J. Mol. Sci. 2020, 21, x FOR PEER REVIEW protein and the E22 envelope protein for expression as a large polyprotein, which will be processed into individual proteins. Introduction of an FMDV-2A protease sequence in the KUN vector will allow removal of remaining KUN flanking regions from the recombinant product [18]. Virus production from KUN vectors has been facilitated by the engineering of a packaging cell line [19]. In addition to KUN vectors, expression systems for West Nile virus [20,21], yellow fever virus [22,23], Int.dengue J. Mol. Sci.virus2020 [24,25], 21, 5130 and tick-borne encephalitis [26,27] have been engineered. 3 of 29 Figure 1. Semliki Forest virus-based expression systems. A. Schematic illustration of expression and helperFigure vectors. 1. Semliki Dark Forest triangle, virus SP6-based RNA expression polymerase systems. promoter; A. lightSchematic triangle, illustration Semliki Forest of expression virus (SFV) and 26Shelper subgenomic vectors. promoter. Dark triangle,B. RNA SP6 replicon RNA (nakedpolymerase RNA promoter; or liposome-encapsulated light triangle, RNA),Semliki recombinant Forest virus virus(SFV particles) 26S subgenomic or DNA replicon promoter. (DNA
Details
-
File Typepdf
-
Upload Time-
-
Content LanguagesEnglish
-
Upload UserAnonymous/Not logged-in
-
File Pages29 Page
-
File Size-