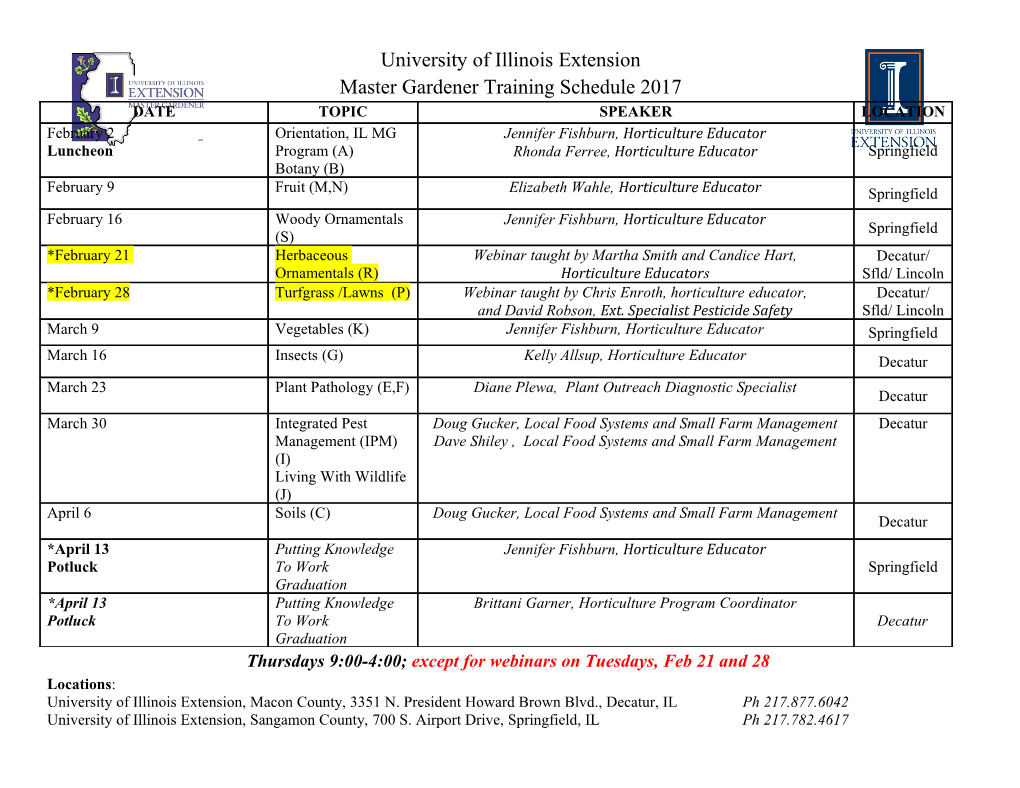
DEVELOPMENT OF METAL-CATALYZED REACTIONS OF ALLENES WITH IMINES AND THE INVESTIGATION OF BRΘNSTED ACID CATALYZED ENE REACTIONS BY LINDSEY O. DAVIS A Dissertation Submitted to the Graduate Faculty of WAKE FOREST UNIVERSITY GRADUATE SCHOOL OF ARTS AND SCIENCES in Partial Fulfillment of the Requirements for the Degree of DOCTOR OF PHILOSOPHY in the Department of Chemistry December 2009 Winston-Salem, North Carolina Approved By: Paul B. Jones, Ph.D., Advisor ____________________________ Examining Committee: Christa L. Colyer, Ph.D., chair ____________________________ S. Bruce King, Ph. D. _______________________________ Dilip K. Kondepudi, Ph.D. _______________________________ Suzanne L. Tobey, Ph.D. _______________________________ ACKNOWLEDGMENTS I must first acknowledge my family for their support throughout my education. My mother has always encouraged me to ask questions and seek answers, which has guided my inquisitive nature as a scientist. My grandparents have taught me the importance of character and my father taught me the value in hard work. I’d also like to thank my husband for moving away from Georgia, a sacrifice I greatly appreciate. Also, his cheerful disposition helped me stay relatively positive, especially when I had bad research days. My friends also played a very important role in keeping me positive during my time at Wake. Particularly my roommates put up with me more than most. I’d like to thank Lauren Eiter for her sense of humor, Tara Weaver for her taste in books and movies, and Jenna DuMond, for her encouragement and loyalty. Also I’ve made lifetime friends with Meredith and Kavita, and even though they left me at Wake, they were always willing to offer their support. I’d also like to thank other people in the department that were a great asset as friends and fellow chemists: Erika Klorig, Ranjan Banerjee, John Solano, Zhidong Ma, Salwa Elkazaz, Lu Rao, Julie Reisz, and Carl Young. My labmates have spent more time with me than anyone else over the course of the past few years. I would like to thank the members of the Tobey and Jones lab for putting up with me and enriching my time at Wake. No one understands what it is like to be a graduate student in the Tobey lab other than Katherine House. We have had some very memorable experiences in the lab II and her persistence encouraged me to finish when I wanted to quit. I have also been very fortunate to have good undergraduates in our lab: Mallory, James, Lauren, and Ritu. James and Lauren in particular assisted on my project, so I thank them for their time and effort. In addition, the members of the Jones lab (Salwa, Saurav, Swapna, Eric) were supportive colleagues and their input was invaluable. I have been fortunate enough to have several amazing chemistry teachers throughout my education. My high school chemistry teacher, Ms. Roberson, gave me a priceless foundation in chemistry, which gave me the initial interest in the subject. My undergraduate career was marked with several great teachers, but none as important as Dr. Gary Breton. Working in his lab not only gave me invaluable lab skills, but it also sparked a love for organic chemistry. At Wake, I would like to first thank my committee members, Dr. Christa Colyer, Dr. Bruce King (especially for stepping in at the last minute), Dr. Welker, and Dr. Kondepudi for reading my thesis and proposals, and for their thoughtful input. I would also like to thank Dr. Marcus Wright not only for his contribution to my education and my project, but also for his spiritual guidance and example. Lastly I would like to thank each of my advisors, Dr. Suzanne Tobey and Dr. Paul Jones. I can’t thank Dr. Tobey enough for her unrelenting dedication to see me through to a doctorate. She is a phenomenal chemist, teacher, and mentor, and I am so glad I was a part of her group. I also want to thank Paul for not only allowing me to join his group, but also his guidance and input to my project. He has been an excellent advisor and teacher. III This dissertation is dedicated in loving memory to Jerel Dale Oliver IV TABLE OF CONTENTS Page List of Tables VIII List of Figures VIII List of Schemes X Abstract XIII Chapter 1 Introduction and Background 1.1.0 Introduction 1 1.2.0 Allenes 2 1.2.1 Synthesis and reactivity of allenes 3 1.2.2 Allenes in the Baylis–Hillman reaction 6 1.3.0 The ene reaction 8 1.3.1 Lewis acid catalysis of the ene reaction 9 1.3.2 Brønsted acid catalysis 11 1.3.3 Brønsted acid catalysis in nature 13 1.3.4 Small molecule organocatalysis 15 1.3.5 Imines and hydrazones 20 1.3.6 Types of ene reactions 22 1.3.6.1 Aza-ene and thio-ene reactions 23 1.3.6.2 The carbonyl-ene reaction 24 1.3.6.3 The imino-ene reaction 29 1.4.0 Unnatural amino acids 31 V 1.5.0 References 33 Chapter 2 Reaction Discovery of allenes and imines 40 2.1.0 Introduction 40 2.2.0 Screening of allenes and imines 41 2.3.0 Optimization of aza-Baylis−Hillman-type reaction 42 2.3.1 Mechanistic studies 46 2.3.2 Other Lewis acids used to promote an alkylation reaction 50 2.3.3 Scope of the reaction 52 2.4.0 Future work 57 2.5.0 Experimental data 60 Chapter 3 Brønsted acid catalyzed ene reactions 81 3.1.0 Introduction 81 3.2.0 Carbonyl-ene reaction 81 3.2.1 Scope of the carbonyl-ene reaction 84 3.2.2 Intramolecular carbonyl-ene reaction 85 3.3.0 Imino-ene reaction 86 3.3.1 Optimization of a Brønsted acid catalyzed imino-ene reaction 87 3.3.2 Scope of the imino-ene reaction 89 3.3.3 1H NMR titration studies of imino-ene reaction 90 3.4.0 Reactions of hydrazones 95 3.4.1 Reaction optimization 97 3.4.2 Scope of the reaction 102 3.4.3. Proposed mechanism and 1H NMR studies 103 VI 3.4.4 Intermolecular hydrazone substrates 107 3.4.5 Future work 108 3.5.0 Experimental Data 109 3.5.1 Carbonyl-ene data 110 3.5.2 Imino-ene data 111 3.5.3 Hydrazone data 119 3.6.0 References 126 Appendix I 129 Appendix II 132 Appendix 136 III Scholastic 149 Vita VII LIST OF TABLES Table I. Optimization of concentration and substrate ratio 44 Table II. Optimization of temperature and time 45 Table III. The effects of additives to probe mechanism 48 Table IV. Other Lewis acids used to promote alkylation of the 51 allene Table V. Scope of the reaction 53 Table VI. Elimination of α-pre-eliminated products 55 Table VII. Styrene optimization results 83 Table VIII. Scope of the carbonyl-ene reaction 84 Table IX. Optimization of imino-ene reaction 88 Table X. Scope of the Brønsted acid catalyzed imino-ene 90 reaction Table XI. Optimization of hydrazone cyclization 98 Table XII. The effect of time on the cyclization reaction 99 Table XIII. The effect of additional Brønsted acids on the cyclization reaction 100 Table XIV. Scope of the reaction 102 Table XV. The effect of water on the cyclization reaction 105 LIST OF FIGURES Figure 1.1 Orbitals and hybridization of allenes. 3 Figure 1.2 Reactivity of donor-substituted allenes. 5 VIII Figure 1.3 HOMO-LUMO interaction between ene and enophile. 8 Figure 1.4 Transition states of thermal and Lewis acid catalyzed 10 ene reactions. Figure 1.5 Activation of carbonyls and imines through hydrogen bonding and metal chelation. 11 Figure 1.6 Thiourea and urea general structures. 15 Figure 1.7 Thiourea dual hydrogen-bond activation of an imine. 16 Figure 1.8 General structures for amidine and guanidine moieties. 16 Figure 1.9 General structures for phosphoric acid, phosphoric acid esters, and phosphoramidates. 18 Figure 1.10 General hydrazone structure. 21 Figure 1.11 Titanium-BINOL dinuclear complex. 26 Figure 1.12 Structure of gymnangiamide. 32 Figure 2.1 Allenes and imines prepared for screening for an allenyl imino-ene reaction. 41 Figure 2.2 Additional allenes tested for an alkyation reaction. 50 Figure 3.1 Structure of diethyl phosphoramidate. 89 Figure 3.2 Graphical representation of the dilution of diethyl phosphate. 91 Figure 3.3 Graphical representation of the titration of tosyl imine into diethyl phosphate. 92 Figure 3.4 Stacked 1H NMR spectroscopy plots of the kinetic experiment of the tosyl imine and diethyl phosphate. 93 Figure 3.5 Graphical representation of the disappearance of the tosyl imine and appearance of ethyl glyoxylate. 94 Figure 3.6 Potential structure of dimerized diethyl phosphate. 95 Figure 3.7 Job plot resulting from the interaction of diethyl phosphate and hydrazone 86 106 IX LIST OF SCHEMES Scheme 1.1 The oxidation of a homopropargylic alcohol. 4 Scheme 1.2 Copper mediated substitution reaction to generate an allene. 4 Scheme 1.3 Pd-catalyzed coupling reaction to generate an allene. 5 Scheme 1.4 General mechanism of the Baylis–Hillman reaction. 6 Scheme 1.5 Aza-Baylis–Hillman between tosylated aldimines and an allene. 7 Scheme 1.6 MgBr2-mediated Baylis–Hillman-type reaction. 8 Scheme 1.7 General ene reaction mechanism. 8 Scheme 1.8 Biradical mechanism of the ene reaction. 9 Scheme 1.9 Step-wise mechanism for a Lewis acid catalyzed ene reaction. 10 Scheme 1.10 General mechanisms for specific acid catalysis and general acid catalysis. 12 Scheme 1.11 Mechanism of serine protease.
Details
-
File Typepdf
-
Upload Time-
-
Content LanguagesEnglish
-
Upload UserAnonymous/Not logged-in
-
File Pages167 Page
-
File Size-