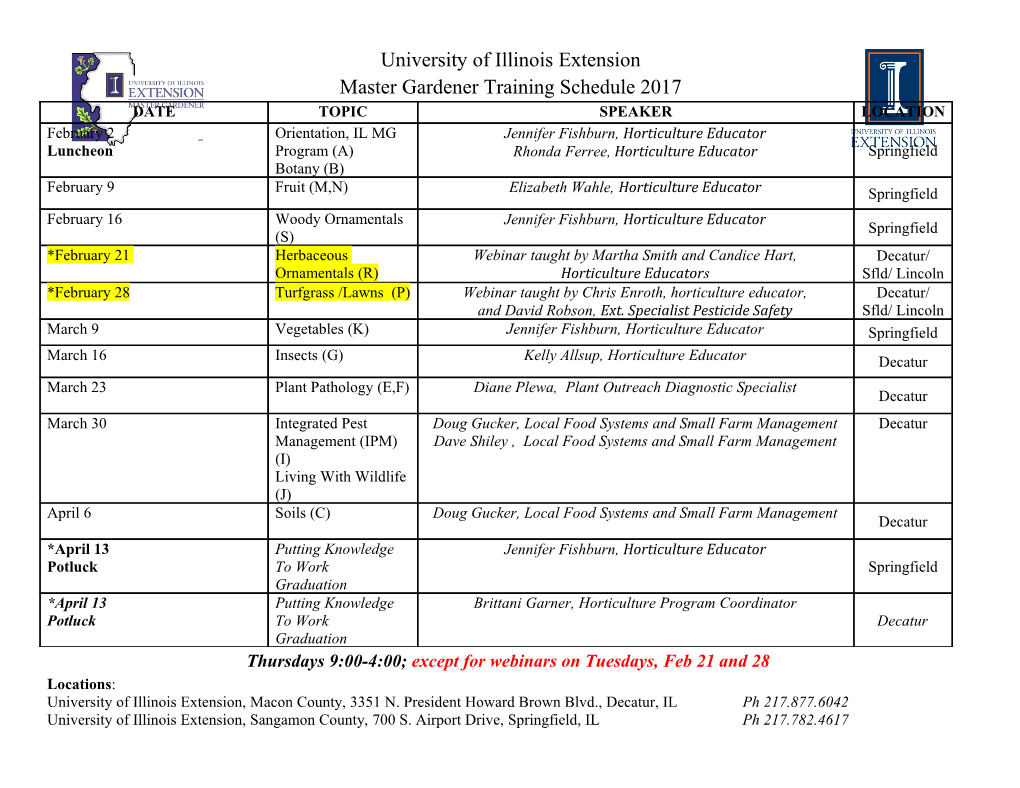
Biogeosciences, 16, 811–829, 2019 https://doi.org/10.5194/bg-16-811-2019 © Author(s) 2019. This work is distributed under the Creative Commons Attribution 4.0 License. Mineral formation induced by cable bacteria performing long-distance electron transport in marine sediments Nicole M. J. Geerlings1, Eva-Maria Zetsche2,3, Silvia Hidalgo-Martinez4, Jack J. Middelburg1, and Filip J. R. Meysman4,5 1Department of Earth Sciences, Utrecht University, Princetonplein 8a, 3584 CB Utrecht, the Netherlands 2Department of Marine Sciences, University of Gothenburg, Carl Skottsberg gata 22B, 41319 Gothenburg, Sweden 3Department of Estuarine and Delta Systems, Royal Netherlands Institute for Sea Research, Utrecht University, Korringaweg 7, 4401 NT Yerseke, the Netherlands 4Department of Biology, Ecosystem Management Research Group, Universiteit Antwerpen, Universiteitsplein 1, 2160 Antwerp, Belgium 5Department of Biotechnology, Delft University of Technology, Van der Maasweg 9, 2629 HZ Delft, the Netherlands Correspondence: Nicole M. J. Geerlings ([email protected]) Received: 10 October 2018 – Discussion started: 22 October 2018 Revised: 10 January 2019 – Accepted: 26 January 2019 – Published: 13 February 2019 Abstract. Cable bacteria are multicellular, filamentous mi- esize that the complete encrustation of filaments might create croorganisms that are capable of transporting electrons over a diffusion barrier and negatively impact the metabolism of centimeter-scale distances. Although recently discovered, the cable bacteria. these bacteria appear to be widely present in the seafloor, and when active they exert a strong imprint on the local geochemistry. In particular, their electrogenic metabolism in- 1 Introduction duces unusually strong pH excursions in aquatic sediments, which induces considerable mineral dissolution, and subse- 1.1 Cable bacteria quent mineral reprecipitation. However, at present, it is un- known whether and how cable bacteria play an active or di- In 2012, long, multicellular microorganisms were reported rect role in the mineral reprecipitation process. To this end from in marine sediments that are capable of generating and we present an explorative study of the formation of sedi- mediating electrical currents over centimeter-scale distances mentary minerals in and near filamentous cable bacteria us- (Pfeffer et al., 2012). These so-called “cable bacteria” have ing a combined approach of electron microscopy and spec- evolved a unique energy metabolism, in which electrons are troscopic techniques. Our observations reveal the formation passed on from cell to cell, thus establishing an electrical cur- of polyphosphate granules within the cells and two different rent from one end to the other of their centimeter-long fila- types of biomineral formation directly associated with mul- mentous bodies. This biological innovation equips cable bac- ticellular filaments of these cable bacteria: (i) the attachment teria with a competitive advantage for survival within the re- and incorporation of clay particles in a coating surround- dox gradients that exist within the seafloor (Meysman, 2017). ing the bacteria and (ii) encrustation of the cell envelope by The electrical connection allows electron donors and elec- iron minerals. These findings suggest a complex interaction tron acceptors to be harvested in widely separated locations between cable bacteria and the surrounding sediment ma- (Nielsen et al., 2010), and in this way the energy yield from trix, and a substantial imprint of the electrogenic metabolism natural redox gradients can be favorably optimized. on mineral diagenesis and sedimentary biogeochemical cy- The currently known cable bacteria strains belong to two cling. In particular, the encrustation process leaves many candidate genera within the Desulfobulbaceae family of open questions for further research. For example, we hypoth- Deltaproteobacteria: the marine “Candidatus Electrothrix” and the freshwater “Candidatus Electronema” (Trojan et Published by Copernicus Publications on behalf of the European Geosciences Union. 812 N. M. J. Geerlings et al.: Mineral formation induced by cable bacteria Figure 1. Microbially and chemically mediated reactions involved in the iron, sulfur and calcium cycle in electrogenic sediments. The electrogenic sulfur oxidation executed by cable bacteria spatially separates the redox half-reactions; the oxidation of sulfide takes place in the suboxic zone and generates electrons (e−). These electrons are then transported through the cable bacteria and used via oxygen reduction. The sulfide oxidation reaction results in proton release and a decrease in pH while the oxygen reduction requires protons, which increases the pH of the porewater in the oxic zone. The decreased pH in the suboxic zone promotes dissolution of iron monosulfides (FeS) and calcium 2C carbonates (CaCO3). The hydrogen sulfide (H2S) produced by iron monosulfide dissolution is used by the cable bacteria. The Fe diffuses both upward and downward. When Fe2C encounters hydrogen sulfide in the sulfidic zone it precipitates again as iron(II) sulfide. In the oxic zone, Fe2C is reoxidized to Fe3C, which will then precipitate as amorphous iron oxide. The calcium ions released by carbonate dissolution diffuse upward and precipitate again as carbonates in the oxic zone where the pH is higher. al., 2016). Cable bacteria perform electrogenic sulfur oxi- imprint suggests that they could be important in the cycling dation (e-SOx) via long-distance electron transport (LDET). of carbon, sulfur, iron and other elements in various natural Thereby, they engender an electrical coupling of the oxida- environments (Nielsen and Risgaard-Petersen, 2015). tion of sulfide within deeper sediments with the reduction of oxygen near the sediment surface (Fig. 1). The anodic 1.2 Acidification and mineral cycling half-reaction in the anoxic zone (half-reaction: 1=2H2S C 2H O ! 1=2SO2− C4e− C5HC) generates electrons, which 2 4 A remarkable aspect of the metabolism of cable bacteria is are then transported along the longitudinal axis of the fila- that they induce unusually strong pH excursions in aquatic ment to cells located in the oxic zone (Pfeffer et al., 2012). In sediments (Nielsen et al., 2010; Meysman et al., 2015). These this thin oxic zone, cathodic cells consume the electrons via large pH excursions are a direct consequence of the spatial oxygen reduction (half-reaction: O C4HC C4e− ! 2H O). 2 2 segregation of redox half-reactions made possible by LDET Field investigations have shown that this separation of redox (Fig. 1). Considerable amounts of protons are released by half-reactions can span a distance up to 7 cm (van de Velde et anodic sulfur oxidation in deeper sediment horizons, thus al., 2016), thus illustrating the wide spatial scale over which strongly acidifying the porewater (down to pH 6 and below). LDET can be active. Conversely, large quantities of protons are consumed by ca- LDET has recently been documented to be active in a thodic oxygen consumption, leading to a characteristic pH wide range of marine environments (Malkin et al., 2014; Bur- maximum in the oxic zone (with pH values up to 9). Due to dorf et al., 2017), including salt marshes, mangroves, sea- these pH excursions in the porewater, the solid-phase chem- grasses and seasonally hypoxic basins, as well as in fresh- istry is also affected by the metabolic activity of the cable water streambeds (Risgaard-Petersen et al., 2015) and pos- bacteria. sibly in aquifer sediments (Müller et al., 2016). LDET has The acidification of the suboxic zone results in the dissolu- furthermore proven to exert a dominant influence on bio- tion of iron(II) sulfide (FeS) and calcium carbonate (CaCO ), geochemical transformation and fluxes in the seafloor. This 3 which causes accumulation of ferrous iron (Fe2C), calcium impact can even extend beyond the sediment and can con- (Ca2C) and manganese (Mn2C) in the porewater (Risgaard- trol the overall cycling of iron, sulfur and phosphorus within Petersen et al., 2012; Rao et al., 2016; Sulu-Gambari et al., the sediment and water column of coastal systems (Seitaj et 2016; van de Velde et al., 2016). Upon release, these cations al., 2015; Sulu-Gambari et al., 2016). The widespread oc- diffuse both upward and downward in the sediment column currence of cable bacteria and their strong local geochemical (Fig. 1). The downward diffusion of Fe2C results in the pre- Biogeosciences, 16, 811–829, 2019 www.biogeosciences.net/16/811/2019/ N. M. J. Geerlings et al.: Mineral formation induced by cable bacteria 813 cipitation of FeS once the ferrous iron encounters the sul- able. Minerals formed by BCM show a high degree of crys- fide appearance depth. The upward diffusion of Fe2C results tallinity and have a specific crystal morphology (Konhauser in the formation of iron (hydr)oxides (FeOOH) once Fe2C and Riding, 2012). reaches the oxic zone (Risgaard-Petersen et al., 2012; Rao et Understanding bacterially mediated mineralization is cru- al., 2016). In a similar manner, Ca2C can diffuse upwards and cial to understanding the complex interactions of biolog- downwards into sediment layers with a higher pH (and car- ical, chemical and physical processes (Konhauser, 1998a; bonate ion concentrations) and reprecipitate as CaCO3, likely Gonzalez-Munoz et al., 2010) since it affects the geochem- in the form of high-magnesium calcite (Risgaard-Petersen et ical cycling of mineral-forming elements (e.g., C, Fe, S, al., 2012). This sometimes gives rise to a hard CaCO3-rich Ca and O) from a
Details
-
File Typepdf
-
Upload Time-
-
Content LanguagesEnglish
-
Upload UserAnonymous/Not logged-in
-
File Pages19 Page
-
File Size-