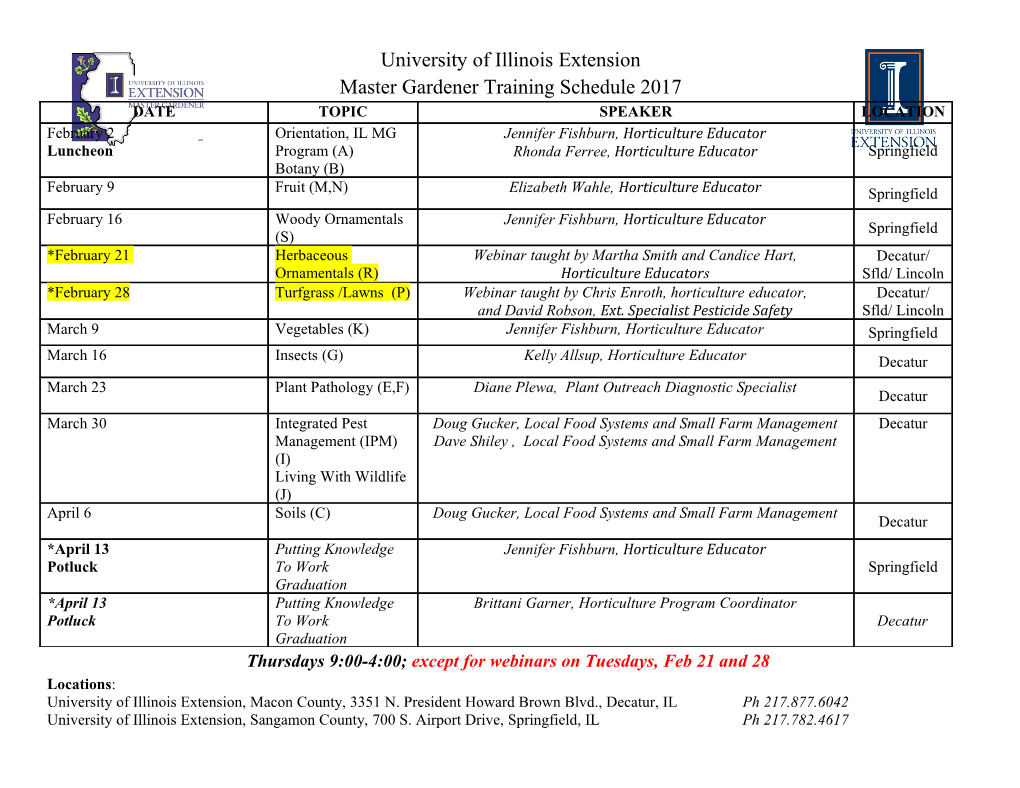
The Plant Journal (2017) 92, 787–795 doi: 10.1111/tpj.13715 Roq1 mediates recognition of the Xanthomonas and Pseudomonas effector proteins XopQ and HopQ1 Alex Schultink, Tiancong Qi, Arielle Lee, Adam D. Steinbrenner and Brian Staskawicz* Department of Plant and Microbial Biology, University of California, Berkeley, CA 94720, USA Received 2 July 2017; revised 30 August 2017; accepted 4 September 2017; published online 11 October 2017. *For correspondence (e-mail [email protected]). SUMMARY Xanthomonas spp. are phytopathogenic bacteria that can cause disease on a wide variety of plant species resulting in significant impacts on crop yields. Limited genetic resistance is available in most crop species and current control methods are often inadequate, particularly when environmental conditions favor dis- ease. The plant Nicotiana benthamiana has been shown to be resistant to Xanthomonas and Pseudomonas due to an immune response triggered by the bacterial effector proteins XopQ and HopQ1, respectively. We used a reverse genetic screen to identify Recognition of XopQ 1 (Roq1), a nucleotide-binding leucine-rich repeat (NLR) protein with a Toll-like interleukin-1 receptor (TIR) domain, which mediates XopQ recognition in N. benthamiana. Roq1 orthologs appear to be present only in the Nicotiana genus. Expression of Roq1 was found to be sufficient for XopQ recognition in both the closely-related Nicotiana sylvestris and the dis- tantly-related beet plant (Beta vulgaris). Roq1 was found to co-immunoprecipitate with XopQ, suggesting a physical association between the two proteins. Roq1 is able to recognize XopQ alleles from various Xan- thomonas species, as well as HopQ1 from Pseudomonas, demonstrating widespread potential application in protecting crop plants from these pathogens. Keywords: plant immunity, plant pathogens, plant disease resistance, Nicotiana benthamiana, Xanthomonas, Pseudomonas, XopQ, HopQ1, NLR, non-host resistance. INTRODUCTION Plant pathogens are a significant problem for agriculture, deliver effector proteins into the plant cell (Rossier et al., resulting in an estimated 10% decrease in crop yields 1999). These effector proteins can function to suppress the despite control measures (Oerke, 2005). Utilizing genetic immune system of the plant or to manipulate the metabo- resistance to protect plants is generally preferable over lism of the host to promote pathogenesis (Gurlebeck€ et al., chemical methods, which can be more expensive and pose 2006; Block et al., 2008; Toruno~ et al., 2016). While effector risks to human or environmental health (Jones et al., 2014; proteins are typically beneficial to the pathogen, if the Vincelli, 2016); however, in many cases genetic resistance plant contains a perception pathway capable of detecting a is not available. The identification of the genetic basis of particular effector protein a strong immune response can disease resistance pathways can allow for the creation of be triggered known as effector-triggered immunity (ETI; resistant crop varieties either through breeding or trans- Alfano and Collmer, 2004; Chisholm et al., 2006; Jones and genic approaches (Dangl et al., 2013; Rodriguez-Moreno Dangl, 2006). An ETI response is often associated with a et al., 2017). As pathogens may evolve to overcome resis- visible cell death response known as the hypersensitive tance mediated by a single resistance gene, it is desirable response. Identification of the genes responsible for speci- to utilize several independent resistance pathways against fic ETI pathways can allow for engineering disease resis- the same pathogen to confer durable resistance. The iden- tance into susceptible crop varieties. tification of resistance genes, particularly those that have Many ETI responses in plants are mediated by a mem- broad specificity across many pathogen species, therefore ber of the nucleotide-binding leucine-rich repeat (NLR) pro- remains a significant focus of molecular plant pathology tein family, with the typical plant genome encoding research. between 100 and 600 of these proteins (Jones et al., 2016). Plant bacterial pathogens in the genus Xanthomonas There are two general models for the activation of an NLR contain a Type III Secretion System, which is used to protein by an effector (Jones and Dangl, 2006). The first is © 2017 The Authors 787 The Plant Journal published by John Wiley & Sons Ltd and Society for Experimental Biology. This is an open access article under the terms of the Creative Commons Attribution License, which permits use, distribution and reproduction in any medium, provided the original work is properly cited. 788 Alex Schultink et al. through physical interaction between the effector protein virulence function (Li et al., 2013b; Teper et al., 2014). As and the cognate NLR receptor involving the formation of a 14-3-3 proteins have a role in the plant immune system protein complex. This mechanism of activation depends (Oh et al., 2010), it has been proposed that one or more of on the physical structure of the effector protein but not on the 14-3-3s are targets of XopQ/HopQ1 and that the viru- its enzymatic activity. Alternatively, NLR recognition can be lence function is achieved through modification, degrada- based on the activity of an effector. In the ‘guard hypothe- tion or sequestration of these proteins (Teper et al., 2014). sis’ (Dangl and Jones, 2001), an NLR protein is activated Both XopQ and HopQ1 are recognized in the plants when it detects perturbations (such as acetylation, cleav- N. benthamiana and Nicotiana tabacum and trigger an ETI age or uridylation) to a specific host factor, typically a pro- response (Wei et al., 2007; Ferrante et al., 2009; Schwartz tein. Because effector perception in the guard model is et al., 2015). The recognition of XopQ/HopQ1 in Nicotiana dependent on the activity and not the physical structure of species is not dependent on interaction with host 14-3-3 the effector protein, this model is generally thought to con- proteins, suggesting that 14-3-3 proteins are not involved vey a resistance that is more difficult to overcome without in the recognition pathway (Li et al., 2013b). Perception of a loss of effector virulence function. XopQ/HopQ1 in N. tabacum is independent of the putative NLR proteins can be divided into groups based on their active site of the protein (Li et al., 2013a; Adlung and domain architecture and genetic dependencies required for Bonas, 2017). The recognition of XopQ and HopQ1 was their function. The two N-terminal domains common on recently reported to be dependent on the EDS1 gene, sug- plant NLR proteins are the Toll-like interleukin-1 receptor gesting a TLR may be involved in the pathway (Adlung (TIR) domain on TIR-NLRs (TLRs) and the coiled coil et al., 2016). In this study we investigated the perception domain on CC-NLRs. All NLR proteins appear to depend pathway of XopQ in N. benthamiana and identified a TLR on the protein SGT1 for function, whereas the TLRs require protein, subsequently named Recognition of XopQ 1 a functional EDS1 protein and a subset of the CC-NLRs in (Roq1), required for perception of XopQ/HopQ1. the Solanaceae require the NRC proteins (Wiermer et al., 2005; Shirasu, 2009; Wu et al., 2016). These genetic depen- RESULTS dencies can be used to help determine which family or Generation of stable Nicotiana benthamiana eds1 mutants clade of genes may be responsible for mediating the per- ception of a particular effector protein. Having a stable knockout of EDS1 in N. benthamiana can The effector protein XopQ from Xanthomonas and the aid in determining whether an unknown immune percep- close-homolog HopQ1 from Pseudomonas are widely dis- tion pathway may be mediated by a TLR and can also be tributed and highly conserved among various species in useful for experiments investigating the mechanism of TLR these genera. XopQ and HopQ1 have been shown to sup- activation. For these reasons we used CRISPR/CAS9 (Jinek press the immune system of the plant and promote patho- et al., 2012) to induce mutations in the EDS1 gene in gen virulence (Li et al., 2013b; Sinha et al., 2013; Hann N. benthamiana. Three guide RNAs were designed and et al., 2014; Teper et al., 2014; Gupta et al., 2015). The tested in vitro to determine if they could mediate CAS9 mechanism by which XopQ suppresses immunity is not cleavage of the target EDS1 gene. All three guides were fully understood and is complicated by the observation active in vitro, and two of the guides were active when that XopQ recognition in Nicotiana benthamiana can sup- expressed transiently from a binary plasmid delivered by press visible cell death responses induced by the expres- Agrobacterium into N. benthamiana (Figure S1). These sion of other pathogen effectors independently from the constructs were used to create stable N. benthamiana virulence activity of XopQ (Adlung and Bonas, 2017). XopQ transformants. Heterozygous mutants were obtained in the has homology to nucleoside hydrolases and a structural T0 population, which were selfed to allow isolation of study suggested that it can hydrolyze a molecule with a homozygous lines. Two independent frameshift deletion ribosyl group (Yu et al., 2014), as demonstrated by in vitro alleles, one for each guide sequence, were obtained and hydrolase activity on the substrate 4-nitrophyenyl b-D-ribo- named eds1-1 and eds1-2 (Figure S1). furanoside (Gupta et al., 2015). A recent study demon- Perception of XopQ depends on EDS1 strated that HopQ1 can hydrolyze the cytokinin precursor iP-riboside 50-monophosphate (iPRMP) in vitro and activate The Xanthomonas effector protein XopQ and the close cytokinin signaling in vivo, suggesting this as the mecha- homolog HopQ1 from Pseudomonas have previously been nism for immune suppression (Hann et al., 2014). An alter- shown to trigger an ETI response in N. benthamiana, indi- native hypothesis is that XopQ/HopQ1 virulence function cating the presence of an immune perception pathway cap- occurs through direct targeting of 14-3-3 proteins.
Details
-
File Typepdf
-
Upload Time-
-
Content LanguagesEnglish
-
Upload UserAnonymous/Not logged-in
-
File Pages9 Page
-
File Size-