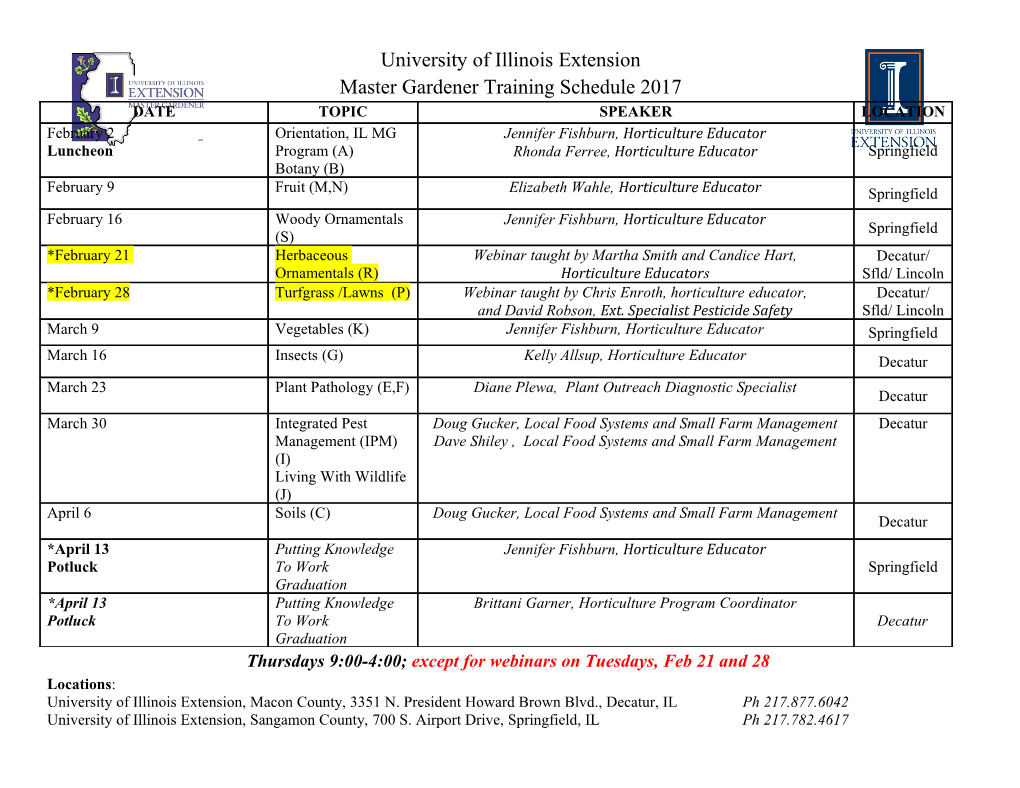
Space Sci Rev DOI 10.1007/s11214-010-9727-y Spectroscopy in Utrecht: A Brief History Frank Verbunt · Johan Bleeker Received: 30 September 2010 / Accepted: 4 November 2010 © The Author(s) 2010. This article is published with open access at Springerlink.com Abstract In the first half of the 20th century, Leonard Ornstein turned the Physics Labora- tory of Utrecht University into a center for testing the nascent quantum mechanics through accurate measurements of line intensities in flame spectra. The microphotometer of instru- ment builder Willem Moll was the key to this success. Marcel Minneart used this instrument to study stellar spectra at the Utrecht Astronomical Institute, and in particular for the Photo- metric Atlas of the Solar Spectrum. When the opportunity came in the 1960s to build instru- ments for research from space, it was natural that Kees de Jager opted for spectroscopy, in X-ray observations of the Sun. The expertise developed in this program allowed the Space Research Organisation of the Netherlands to be a principal player in the development of X-ray spectrographs on the Einstein, EXOSAT, Chandra and XMM-Newton satellites. Keywords History of physics · History of astronomy · Instrumentation: spectrographs 1 Introduction At the start of a meeting on high-resolution X-ray spectroscopy in Utrecht, it is perhaps not inappropriate to have a brief look at the history of spectroscopy in Utrecht. The first part of this paper is about optical spectroscopy in the Utrecht physics department from the end of the 19th century to the period in the 1930s when the work by Ornstein and his group had a pronounced impact on the development of quantum mechanics. Our discussion of this period is based mainly on the PhD thesis (in Dutch) by Heijmans (1994). The second part describes the continuation of this work at the Astronomical Institute, and is based mainly on F. Verbunt () · J. Bleeker Astronomical Institute Utrecht University, Utrecht, The Netherlands e-mail: [email protected] J. Bleeker e-mail: [email protected] F. Verbunt · J. Bleeker SRON Netherlands Institute for Space Research, Utrecht, The Netherlands F. Verbunt, J. Bleeker a biography (also in Dutch) of Minnaert, by Molenaar (2003). The final part describes the change from optical to X-ray spectroscopy at the Utrecht branch of the Netherlands Institute for Space Research. 2 Quantitative Spectroscopy for Quantum Mechanics 2.1 Origins: Buys Ballot and Julius In 1863 the Dutch government passed a law on the education system which was to have a deep and lasting impact on the study of the natural sciences in the Netherlands, by es- tablishing a new type of secondary education: the Hogere Burger School (higher school for citizens), the HBS. The HBS emphasized education in the natural sciences, and provided the universities with students from middle and lower income groups who in earlier times would not have had higher education. The HBS directly lead to a flowering of the natural sciences in the Netherlands, as witnessed by a number of Dutch Nobel-Prize laureates in physics and chemistry in the early 20th century (Willink 1998). The first university students who arrived from the HBS discovered to their surprise that the experimental labs at the universities were outdated with respect to the high-school labo- ratories, and in Utrecht Buys Ballot, who in 1853 had founded the Sonnenborgh Observatory for meteorology and astronomy, founded a new physics laboratory, devoted mainly to teach- ing, in 1877. As an intermezzo we may mention here the influence that Buys Ballot had on the career of a certain Wilhelm Conrad Röntgen, a youngster who refused to tell on prank of a fellow student at the Lower Technical School, and as a result was thrown out. Thus without a diploma, he would not be able to obtain a degree at Utrecht University when he arrived there in 1865. Buys Ballot was impressed sufficiently with the qualities of his pupil to write a recommendation letter for him to the ETH in Zürich, Switzerland, and so set Röntgen on track for his career. In 1888 Buys Ballot asked W.H. Julius, one of his students in the new laboratory, to study the heat radiation of solids, in particular the spectral energy distribution, following in the footsteps of Kirchhoff and Wien. Hoping to explain the spectra of solids by the emission properties of their basic building blocks, Julius also measured flame spectra of molecules. In 1896 Julius became the director of the physics laboratory and shifted his interest to radiation of the Sun. In these pre-quantum-mechanics days he devised a theory to explain the Fraun- hofer lines in the solar spectrum with anomalous dispersion, and to measure these lines he built a heliostat spectrograph on the roof of the laboratory. Together with astronomers Nijland and Van der Bilt of the Astronomical Observatory in Utrecht, Julius also organized eclipse expeditions, for example to Maastricht in 1912, to measure chromospheric lines. 2.2 The Microphotometer and Quantum Numbers Most measurements of the heat radiation were made with thermocouples. The brilliant in- strument builder Willem Jan Henry Moll in the course of many years perfected the mea- suring apparatus into Moll’s microphotometer, as it became known, the work horse of the spectroscopic work done in Utrecht in the 1920s and beyond (van Cittert 1926;Moll1921, 1926). Moll started by improving the thermocouples by better insulation and by better se- lection of metals. He then attached a mirror to the galvanometer and used a beam of light reflecting on it to magnify the excursion and register it on a slowly moving photographic plate, thus achieving automatic registration. Spectroscopy in Utrecht: A Brief History Fig. 1 Moll’s microphotometer in 1919. For explanation see text Figure 1 shows Moll’s microphotometer as it was in 1919. The light beam passes from its source on the right via a lens through the photographic plate on which the spectrum is recorded onto the thermocouple on the left. This photographic plate is mounted in a frame, and the motion of the frame is mechanically coupled to the motion of a new photographic plate that records the beam reflected from the mirror on the thermocouple. Thus the spectrum could be scanned automatically, as the density (i.e. measure of darkness) on the photographic plate with the spectrum is converted into a tracing (excursion) on the new photographic plate. Julius was succeeded as director of the physics laboratory in 1918 by Leonard Salomon Ornstein. Although trained as a theoretical physicist, he turned the Utrecht institute into a mainly experimental laboratory. He contacted industry to align the experimental physics with its needs, thereby enhancing the level of both industry and the physics laboratory. (Some 20 of Ornsteins 94 (!) graduate students would find work in the Philips Physics Lab- oratory, 11 at the Bataafsche Petroleum Maatschappij.) In fundamental physics, Ornstein decided to contribute to the new physics of quantum mechanics, by measuring not just the wavelengths of spectral lines—which many people were doing—but also the intensities— for which Utrecht was in a unique position. He therefore started calibrating photographic plates from different sources, testing their stability. He introduced the practice of illumi- nating with the light of a calibrated light source a part of each photographic plate which was used to obtain a flame spectrum, thus allowing accurate calibration of each individual plate. He used a stepped wedge, made by depositing platinum in six different thicknesses onto a 2 cm glass plate, to calibrate a large range of intensities at all colours simultaneously. He improved the stability of the thermocouple that served as the calibrating light source. In 1927 the Rockefeller Foundation donated a for those days very large Rowland grating: 13 × 13 cm, with 80 000 lines and a radius of the Rowland circle of R = 5.65 m. Already before this, Ornsteins students Burger and Dorgelo (1924) had formulated sum- mation rules linking quantum numbers of spectral lines, on the basis of relative line strengths (Table 1). These results forced the theoreticians to rethink the rules derived by Sommerfeld and Heisenberg (1922), which predicted intensities proportional to j 2, since the observa- tions indicated a proportionality to j(j + 1). This was an important result as it showed that the transition probability depended on both initial and final state. This required a reformula- tion of the correspondence principle, although it was not obvious how, as witnessed by the following two points of view: F. Verbunt, J. Bleeker Table 1 Conversion of experimentally determined relative intensities of calcium multiplet lines into gener- alized summation rules, after Burger and Dorgelo (1924) Measurements calcium multiplet (rounded) p1–d3 p1–d2 p1–d1 p2–d3 p2–d2 p3–d3 1 18 100 19 54 25 Converted to tabular form J = 2j + 1 d1(7/2) d2(5/2) d3(3/2) Sum p1(5/2) 100 18 1 119 p2(3/2) – 54 19 73 p3(1/2) – – 25 25 Sum 100 72 45 Generalized theory version 2k + 32k + 12k − 1Sum 2k + 112k2 + 12k − 9 u 12 − u(2k + 1)(6k + 3) 2k − 1– 12k2 − 3 − uu (2k − 1)(6k + 3) 2k − 3– – 12k2 − 12k − 9 (2k − 3)(6k + 3) Sum (2k + 3)(6k − 3)(2k + 1)(6k − 3)(2k − 1)(6k − 3) The fundamental intensity-measurements of Ornstein etc. show that reality is much simpler and more arithmetical than expected from the Correspondence Principle. (Sommerfeld, letter to Kramers 1924) As regards the “intensities a la Ornstein” I fully share your view...BohrandI have consideredthiscarefullyoncemoreandconcludedthatthesummationrules...follow unavoidably from the correspondence principle. (Heisenberg, letter to Pauli 1924) Note that the measurements of Ornstein were sufficiently known that Sommerfeld and Heisenberg did not need to specify them.
Details
-
File Typepdf
-
Upload Time-
-
Content LanguagesEnglish
-
Upload UserAnonymous/Not logged-in
-
File Pages11 Page
-
File Size-