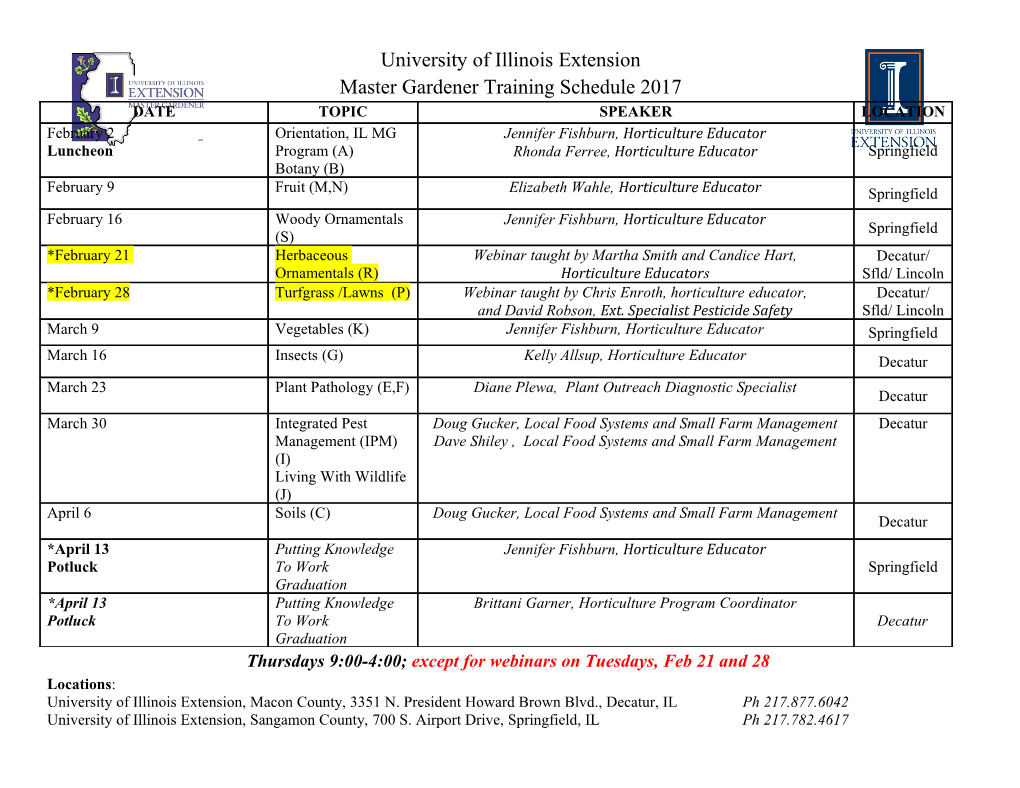
Atmos. Chem. Phys., 4, 1355–1363, 2004 www.atmos-chem-phys.org/acp/4/1355/ Atmospheric SRef-ID: 1680-7324/acp/2004-4-1355 Chemistry and Physics Diurnal and annual variations of meteor rates at the arctic circle W. Singer, U. von Zahn, and J. Weiß Leibniz-Institute of Atmospheric Physics, 18225 Kuhlungsborn,¨ Germany Received: 15 October 2003 – Published in Atmos. Chem. Phys. Discuss.: 6 November 2003 Revised: 28 July 2004 – Accepted: 28 July 2004 – Published: 23 August 2004 Abstract. Meteors are an important source for (a) the metal 1 Introduction atoms of the upper atmosphere metal layers and (b) for con- densation nuclei, the existence of which are a prerequisite A meteoroid is a small particle travelling in interplanetary for the formation of noctilucent cloud particles in the polar space. In the case that it enters the Earth’s upper atmosphere mesopause region. For a better understanding of these phe- its interactions with the air molecules give rise to an extended nomena, it would be helpful to know accurately the annual trail of ionization (detectable by radars) and, if the meteoroid and diurnal variations of meteor rates. So far, these rates is large and fast enough, to a brief streak of light (the visible have been little studied at polar latitudes. Therefore we have meteor). As common in the literature, we will apply the des- used the 33 MHz meteor radar of the ALOMAR observatory ignation ‘meteor’ in the following also to the radar-detected at 69◦ N to measure the meteor rates at this location for two ◦ trail of ionization. The ablation of meteoroids during their full annual cycles. This site, being within 3 of the Arctic high-speed entry into the Earth’s atmosphere is an important circle, offers in addition an interesting capability: The axis source for (a) the metal atoms of the upper atmosphere metal of its antenna field points (almost) towards the North eclip- layers and (b) also for the formation of nanometer-sized con- tic pole once each day of the year. In this particular viewing densation nuclei (CN). The existence of these CNs is a pre- direction, the radar monitors the meteoroid influx from (al- requisite for the subsequent formation of noctilucent cloud most) the entire ecliptic Northern hemisphere. particles in the polar mesopause region. For a better un- We report on the observed diurnal variations (averaged derstanding of these phenomena, it is helpful to know ac- over one month) of meteor rates and their significant alter- curately the annual and diurnal variations of meteor rates. ations throughout the year. The ratio of maximum over min- These rates have been extensively studied at mid and low lat- imum meteor rates throughout one diurnal cycle is in Jan- itude, starting with the imposing work of Hawkins (1956) at uary and February about 5, from April through December Jodrell Bank (53◦ N). Among his many results are the con- 2.3±0.3. If compared with similar measurements at mid- clusions that the space density of meteors along the length of latitudes, our expectation, that the amplitude of the diurnal the Earth’s orbit clearly peaks in June and that the radiants variation is to decrease towards the North pole, is not really of the sporadic meteors are concentrated towards the plane borne out. of the ecliptic. In comparison, few studies have been pub- Observations with the antenna axis pointing towards the lished on meteor rates and their temporal variations at polar North ecliptic pole showed that the rate of deposition of me- latitudes. Therefore we have integrated a 33 MHz SKiYMET teoric dust is substantially larger during the Arctic NLC sea- all-sky meteor radar into the instrument cluster of the ALO- son than the annual mean deposition rate. The daylight me- MAR observatory at 69◦ N latitude and have measured the teor showers of the Arietids, Zeta Perseids, and Beta Tau- radar meteor rates at this location for two full annual cycles. rids supposedly contribute considerably to the June maxi- This site, being within 3◦ of the Arctic circle, offers in ad- mum of meteor rates. We note, though, that with the radar dition an interesting capability: The axis of its antenna field antenna pointing as described above, all three meteor radi- points (almost) towards the North ecliptic pole (=NEP) once ants are close to the local horizon but all three radiants were each day of the year. In this particular viewing direction, the detected. radar monitors the meteoroid influx from (almost) the entire ecliptic Northern hemisphere. Hence, the radar is excellently Correspondence to: W. Singer suited to measure the spatial variations of that meteoroid in- ([email protected]) flux, which originates in the ecliptic Northern hemisphere, © European Geosciences Union 2004 1356 W. Singer et al.: Meteor rates Fig. 1. Two-element crossed receive antenna of the SKiYMET radar. Panel (a): Horizontal radiation pattern with azimuthal cuts at 10◦, 60◦ and 80◦ zenith angle; Panel (b): Vertical radiation pattern with zenithal cuts at 0◦ and 45◦ azimuth and a 3-dB beam width of 109◦ (courtesy of Genesis Software). along the path of the Earth about the Sun. Here we report on tion from the radar to the meteor trail. Still, the gain pattern the observed diurnal variations (averaged over one month) of implies a rather well behaved detection efficiency for mete- meteor rates and their significant alterations throughout the ors from all directions except for those entering close to the year vertical. Meteor entry velocities can be estimated from about 5% of the detections using the phase oscillations prior and after the 2 The radar instrument: its parameters and sites peak in the signal amplitude and a radiant determination is possible on a statistical basis (for details see Hocking et al., This study was performed using a commercially produced 2001). SKiYMET all-sky interferometer meteor radar (Hocking A theoretical estimate for the limiting meteor sensitivity et al., 2001). Its basic instrument parameters are: of the radar is obtained using the minimum detectable power P at the antenna determined from a sky noise map for frequency 32.55 MHz noise 45 MHz at 69◦ N (Campistron et al., 2001) after Eqs. (1) and peak power 12 kW (2), where k is Boltzman’s constant. pulse width 13 µs pulse rep. frequency 2144 Hz Pnoise = (TA + Tr )kB = {TA + T0(F − 1)} (1) transmitting antenna 3-element crossed Yagi antenna = = −2.5 receiving antenna 5-channel interferometer TA T32.55 MHz T45 MHz(32.55/45) (2) of 2-element crossed Yagi antennas We use the bandwidth of the receiver, B=50 kHz, and sampling resolution 0.94 ms correct also for the small additional receiver noise at height range 78–120 km ◦ T0=290 K with its noise figure, F =4. Using a sky tem- angular resolution 2 −14 perature TA=8000 K a value of Pnoise=1.3·10 W results. Only echoes about 10 dB above the cosmic noise floor will The radar antennas apply crossed antenna elements to en- be detected, so the practical detection threshold power is −13 sure a near uniform azimuthal sensitivity to meteor echoes as Pth=1.3·10 W. For a peak power Pt of 12 kW and a ca- shown in Fig. 1a for the receiver antenna. The system uses ble loss of about 1 dB, a radar frequency f , the speed of light a 5-antenna interferometer on reception, resulting in a range c, and an antenna directivity (D=5.4) at a minimum range R accuracy of 2 km and angular accuracy of 1 to 2◦ in meteor of 80 km, the minimum threshold power corresponds accord- location. The dependence of the receiver antenna gain on the ing to Eq. (3) to a minimum detectable electron line density 12 elevation is shown in Fig. 1b. It is rather uniform for zenith of qlim=3.6·10 electrons/m (McKinley, 1961). ◦ angles less than, say, 60 and tends towards zero for echoes 3 −32 2 3 1/2 qlim = {(PthR )/(2.5 · 10 Pt D (c/f ) )} (3) close to the local horizon. We note that the system detects only meteor trails oriented perpendicular to the radial direc- M = 40 − 2.5 log qlim (4) Atmos. Chem. Phys., 4, 1355–1363, 2004 www.atmos-chem-phys.org/acp/4/1355/ W. Singer et al.: Meteor rates 1357 12000 1.00 10000 54°N 69°N 8000 0.10 6000 decay time [s] meteors/day 4000 2000 0.01 February 2002 0 110 June 2002 01-Jan-00 01-Jan-01 01-Jan-02 01-Jan-03 01-Jan-04 100 Fig. 2. Annual variation of daily mean meteor count rates at Julius- ◦ ◦ ruh (54 N) and Andenes (69 N) between November 1999 and 90 height [km] April 2004. 80 70 This qlim corresponds to a meteor of radio magnitude +8.6 0.0 0.2 0.4 0.6 0.8 1.0 using the relation between magnitude and electron line den- frequency of occurrence sity given by Eq. (4). From November 1999 until August 2001, the radar was Fig. 3. Monthly mean distributions of the meteor occurrence at operated at our field station Juliusruh, Germany, with ge- Andenes. The peak rates are 9271 meteors in February 2002 and ◦ ◦ ographic coordinates 54.6 N, 13.4 E. Thereafter the radar 47 753 meteors in June 2002. was moved to the ALOMAR observatory, Norway, with geographic coordinates 69.3◦ N, 16.0◦ E (von Zahn, 1997). There the radar is located 2.6◦ N of the Arctic circle and is in continuous operation since the end of September 2001 (Singer et al., 2003).
Details
-
File Typepdf
-
Upload Time-
-
Content LanguagesEnglish
-
Upload UserAnonymous/Not logged-in
-
File Pages9 Page
-
File Size-