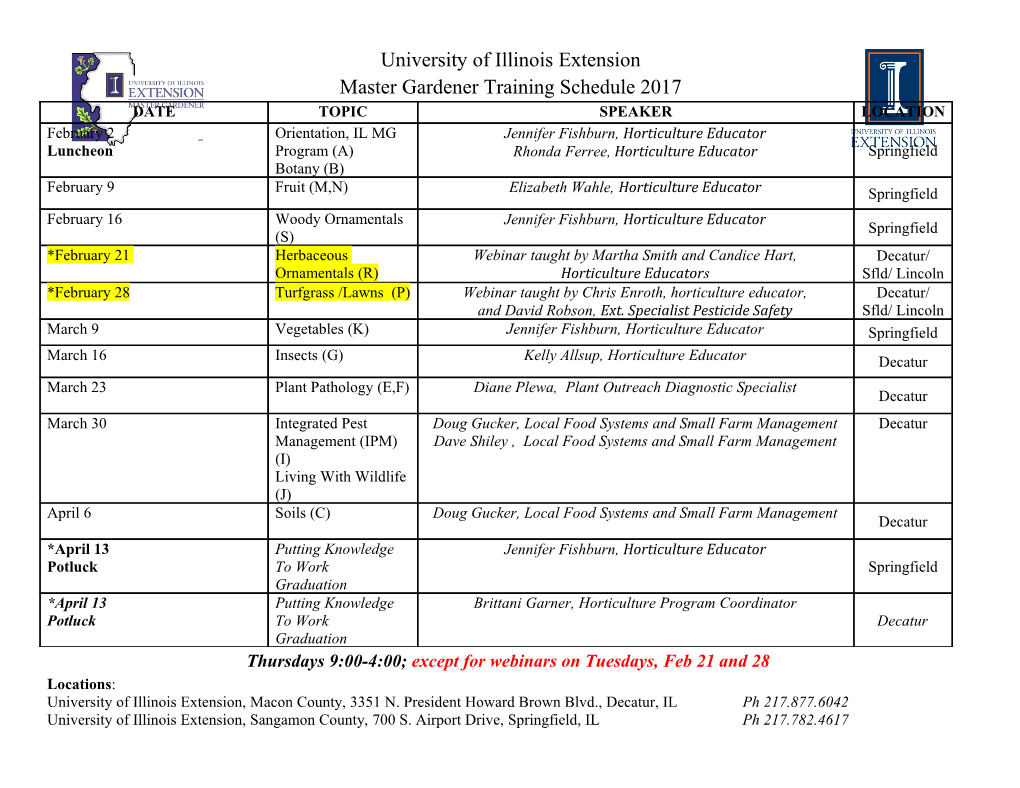
THESIS SPECTRAL GAMMA RAY CHARACTERIZATION OF THE ELKO FORMATION, NEVADA—A CASE STUDY FOR A SMALL LACUSTRINE BASIN Submitted by Erin M. McGowan Department of Geosciences In partial fulfillment of the requirements For the Degree of Master of Science Colorado State University Fort Collins, Colorado Summer 2015 Master’s Committee: Advisor: Sven O. Egenhoff Derek Schutt Gamze Cavdar Robert Amerman Copyright by Erin M. McGowan 2015 All Rights Reserved ABSTRACT SPECTRAL GAMMA RAY CHARACTERIZATION OF THE ELKO FORMATION, NEVADA—A CASE STUDY FOR A SMALL LACUSTRINE BASIN Handheld gamma ray spectrometry is a cost-effective and time-efficient means of furthering understanding of lake facies and small-scale lake systems. Spectral and total gamma ray data were recorded every foot vertically through a succession in situ at four outcrops along a NNW- to-SSE transect representing the lower to middle Eocene Elko Formation in northeast Nevada, USA. The lacustrine Elko Formation consists of, from oldest to youngest, four major units: 1) a basal conglomerate, 2) an overlying carbonate, 3) a fine-grained organic-rich mudstone with intercalated carbonate mudstones, and 4) volcaniclastics. These units comprise fourteen sedimentological facies identifiable in outcrop. In this study, these fourteen facies have been reduced to eight that are discernable by spectral gamma ray (SGR) signals. Each recorded interval in the Elko Formation succession was assigned to one of these eight facies. These eight facies comprise five siliciclastic (plant-bearing mudstone, clay-dominated mudstone, microbial-mat- bearing mudstone, ash-bearing mudstone, and conglomerate) facies, two carbonate (calcareous mudstone and fossiliferous mudstone-wackestone) facies, and one volcanic tuff facies. In conjunction with SGR, outcrop observation, X-ray diffraction (XRD), thin section observation, and total organic carbon (TOC) analyses allowed a thorough understanding of facies composition and its SGR signal. The primary controls of SGR components [potassium (K), uranium (U), and thorium (Th)] reflect K-bearing volcanic minerals (feldspars and micas), U- enriched organic material, and clay abundance (illite and montmorillonite; potentially derived ii from volcanic ash), respectively. High radioactivity, with signals above 120 American Petroleum Institute (API) units, was demonstrated for five facies (plant-bearing mudstone, clay-dominated mudstone, microbial-mat-bearing mudstone, ash-bearing mudstone, and volcanic tuff) in contrast to the remaining three facies (calcareous mudstone, fossiliferous mudstone-packstone, and conglomerate) exhibiting low radioactivity of less than or equal to 120 API. Distribution of radioactive minerals across the outcrops was largely found to be not only a function of general lithologic composition, but also the paleogeographic locations of the outcrops within the lake basin, due to the differing contributions of organic debris and volcanic constituents. This distribution of radioactive minerals across each outcrop supports a recent depositional model of north-to-south diachronous deposition of the Elko Formation (Horner, 2015). The microbial-mat-bearing mudstone facies was a traceable, deep-lake sediment throughout the basin by which outcrops could be assigned to more proximal or more distal positions within the paleo-lake by applying Th/U ratios. The proximal outcrop data show a low Th/U ratio (below 2.5), as opposed to the distal outcrop data that have a high Th/U ratio (2.5–4). These data confirm that most likely Th/U ratios reflect the increase in the amount of clay with distance from the proximal outcrops. Thin section microscopy and scanning electron microscope (SEM) analyses allowed for recognition of eight diagenetic cements reflecting a strongly varying diagenetic history in the Elko Formation sedimentary rocks. One calcite, four stages of dolomite, two types of silica, and one zeolite cement were identified. The calcite, dolomite, and silica cements were typically formed sequentially as listed above, whereas the zeolites formed independently. Intraparticle, interparticle, matrix, shelter, and fracture porosity types are also present, each forming post- deposition, except for interparticle and matrix porosity within volcaniclastics. Calcite, dolomite, iii and silica cements were found succeeding shelter and fracture porosity, whereas the timing of zeolite cement in matrix porosity was unclear. Overall, cement phases and porosity were found to be minimal, and therefore, probably had only a minor influence on the overall gamma ray signal of the Elko Formation sedimentary rocks. SGR characterization of each of the eight facies across a proximal-to-distal transect of the Elko continental-lacustrine sedimentary basin reflects the strong influence that climate and tectonics have on depositional changes in a small-scale lake. Applying gamma ray techniques to the small-scale lake system of the Elko Formation was found to be a useful tool and provides a framework to apply to lacustrine studies as a predictive tool in future exploration. iv ACKNOWLEDGEMENTS I’m thankful to my advisor, Dr. Sven Egenhoff for the many hours of editing, reviewing, and feedback on this study, as well as helping to improve my writing. I’m incredibly grateful to Noble Energy for providing financial support to carry out this research over the past two years —as well as the feedback and genuine interest in the project: Thank you Rob Amerman, Morgan Hocker, Donald Burch, Rick Stucker, and Wendell Bond. From the last field season, thanks to Jon Bowman for arranging a track-hoe for field excavations. I would also like further thank Donald Burch for the time he spent to helping guide this project and giving me insightful suggestions. Many thanks to Heather Lowers at the USGS for her assistance with the SEM and EDS analyses as well as Joe Taglieri from Atoka Geochemical for his XRD services. Each greatly enhanced this work and are much appreciated. I’m also thankful to the Tomera family for allowing us to study the Elko succession on their property and even to excavate the outcrop. Will Horner, Sven Egenhoff, and Rob Amerman, thank you for the enthusiasm and help in the field. I would also like to express my gratitude to my committee members, Dr. Sven Egenhoff, Dr. Gamze Cavdar, and Dr. Rob Amerman for their comments and suggestions to improve the quality of my thesis. CSU sedimentology group, thank you for your support and laughs to make this a great place to work. Finally, a big thank you to my family and Travis Moss for their love, continuous support, and patience. v TABLE OF CONTENTS ABSTRACT .................................................................................................................................... ii ACKNOWLEDGEMENTS ............................................................................................................ v 1.0 INTRODUCTION .................................................................................................................... 1 2.0 GEOLOGICAL SETTING ....................................................................................................... 5 2.1 Regional Tectonism and Volcanism ..................................................................................... 5 2.2 Paleogeography and Paleontology ........................................................................................ 7 2.3 Basin Extents and Shape ....................................................................................................... 7 2.4 Stratigraphy ........................................................................................................................... 8 3.0 REVIEW OF PREVIOUS LITERATURE ............................................................................. 12 3.1 Elko Formation ................................................................................................................... 12 3.2 Gamma Radiation and Spectral Gamma Ray: Potassium, Thorium, and Uranium ............ 21 3.3 Gamma Ray Spectrometer Measurements .......................................................................... 23 4.0 METHODOLOGY ................................................................................................................. 25 4.1 Study Area .......................................................................................................................... 25 4.2 Gamma Ray Spectrometer Tool.......................................................................................... 26 4.3 Field Methods ..................................................................................................................... 27 4.4 Thin section, Total Organic Carbon, X-Ray Diffraction/Fluorescence .............................. 30 5.0 RESULTS ............................................................................................................................... 32 5.1 Total Gamma Ray Data ...................................................................................................... 32 5.2 Spectral Gamma Ray Measurements .................................................................................. 36 5.2.1 Facies 1/2: Plant-Bearing Mudstone (Fig. 13A) .......................................................... 39 5.2.2 Facies 3: Clay-Dominated Mudstone (Fig. 13B) ......................................................... 39 5.2.3 Facies 4: Calcareous Mudstone (Fig. 13C) .................................................................. 39 5.2.4 Facies 5: Microbial-Mat-Bearing-Mudstone
Details
-
File Typepdf
-
Upload Time-
-
Content LanguagesEnglish
-
Upload UserAnonymous/Not logged-in
-
File Pages193 Page
-
File Size-