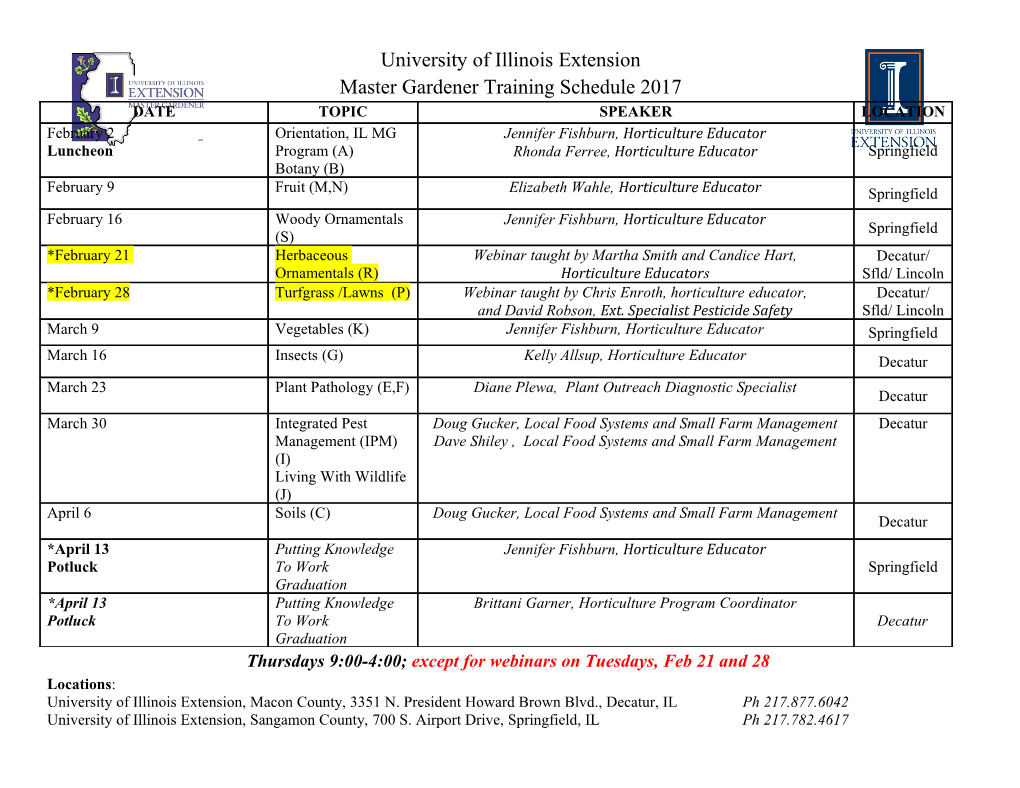
NOTES ON RIEMANN’S ZETA FUNCTION DRAGAN MILICIˇ C´ 1. Gamma function 1.1. Definition of the Gamma function. The integral ∞ Γ(z)= tz−1e−tdt Z0 is well-defined and defines a holomorphic function in the right half-plane {z ∈ C | Re z > 0}. This function is Euler’s Gamma function. First, by integration by parts ∞ ∞ ∞ Γ(z +1)= tze−tdt = −tze−t + z tz−1e−t dt = zΓ(z) Z0 0 Z0 for any z in the right half-plane. In particular, for any positive integer n, we have Γ(n) = (n − 1)Γ(n − 1)=(n − 1)!Γ(1). On the other hand, ∞ ∞ Γ(1) = e−tdt = −e−t = 1; Z0 0 and we have the following result. 1.1.1. Lemma. Γ(n) = (n − 1)! for any n ∈ Z. Therefore, we can view the Gamma function as a extension of the factorial. 1.2. Meromorphic continuation. Now we want to show that Γ extends to a meromorphic function in C. We start with a technical lemma. Z ∞ 1.2.1. Lemma. Let cn, n ∈ +, be complex numbers such such that n=0 |cn| converges. Let P S = {−n | n ∈ Z+ and cn 6=0}. Then ∞ c f(z)= n z + n n=0 X converges absolutely for z ∈ C − S and uniformly on bounded subsets of C − S. The function f is a meromorphic function on C with simple poles at the points in S and Res(f, −n)= cn for any −n ∈ S. 1 2 D. MILICIˇ C´ Proof. Clearly, if |z| < R, we have |z + n| ≥ |n − R| for all n ≥ R. Therefore, we 1 1 have | z+n |≤ n−R for |z| < R and n ≥ R. It follows that for n0 > R, we have ∞ ∞ ∞ ∞ cn |cn| |cn| 1 ≤ ≤ ≤ |cn|. z + n |z + n| n − R n0 − R n=n0 n=n0 n=n0 n=n0 X X X X cn Hence, the series n>R z+n converges absolutely and uniformly on the disk {z | |z| < R} and defines there a holomorphic function. It follows that ∞ cn P n=0 z+n is a meromorphic function on that disk with simple poles at the points of S in ∞ cn P {z | |z| < R}. Therefore, n=0 z+n is a meromorphic function with simple poles at the points in S. Therefore, for any −n ∈ S we have P c c c f(z)= n + m = n + g(z) z + n z + m z + n −m∈XS−{n} where g is holomorphic at −n. This implies that Res(f, −n)= cn. Going back to Γ, we have ∞ 1 ∞ Γ(z)= tz−1e−t dt = tz−1e−t dt + tz−1e−t dt. Z0 Z0 Z1 Clearly, the second integral converges for any complex z and represents an entire function. On the other hand, since the exponential function is entire, its Taylor series converges uniformly on compact sets in C, and we have 1 1 ∞ (−1)p tz−1e−t dt = tz−1 tp dt p! 0 0 p=0 ! Z Z X ∞ (−1)p 1 ∞ (−1)p 1 = tp+z−1 dt = p! p! z + p p=0 0 p=0 X Z X for any z ∈ C. Therefore, ∞ ∞ (−1)p 1 Γ(z)= tz−1e−t dt + p! z + p 1 p=0 Z X for any z in the right half-plane. By 1.2.1, the right side of this equation defines a meromorphic function on the complex plane with simple poles at 0, −1, −2, −3, − · · ·. Hence, we have the following result. 1.2.2. Theorem. The function Γ extends to a meromorphic function on the complex plane. It has simple poles at 0, −1, −2, −3, ···. The residues of Γ are −p are given by (−1)p Res(Γ, −p)= p! for any p ∈ Z+. This result combined with the above calculation immediately implies the follow- ing functional equation. 1.2.3. Proposition. For any z ∈ C we have Γ(z +1)= zΓ(z). NOTESONRIEMANN’SZETAFUNCTION 3 1.3. Another functional equation. Let Re z > 0. Then ∞ Γ(z)= tz−1e−tdt. Z0 Let Re p> 0 and Re q> 0. Then, by change of variable t = u2, we get ∞ ∞ 2 Γ(p)= tp−1e−tdt =2 e−u u2p−1du. Z0 Z0 Analogously we have ∞ 2 Γ(q)=2 e−v v2q−1dv. Z0 Hence, it follows that ∞ ∞ 2 2 Γ(p)Γ(q)=4 e−(u +v )u2p−1v2q−1du dv, Z0 Z0 and by passing to the polar coordinates by u = r cos ϕ, v = r sin ϕ, we have ∞ π 2 2 Γ(p)Γ(q)=4 e−r r2(p+q)−1 cos2p−1 ϕ sin2q−1 ϕ dr dϕ Z0 Z0 ∞ π 2 2 = 2 e−r r2(p+q)−1 dr · 2 cos2p−1 ϕ sin2q−1 ϕ dϕ Z0 Z0 ! π 2 = 2Γ(p + q) cos2p−1 ϕ sin2q−1 ϕ dϕ. Z0 We put s = sin2 ϕ in the integral. Then we have π 2 1 2 cos2p−1 ϕ sin2q−1 ϕ dϕ = sq−1(1 − s)p−1ds. Z0 Z0 If we define 1 B(p, q)= sp−1(1 − s)q−1ds Z0 for Re p> 0, Re q> 0, we get the identity Γ(p)Γ(q) B(p, q)= B(q,p)= . Γ(p + q) Here B is Euler’s Beta function. Let x ∈ (0, 1). Then we have Γ(x)Γ(1 − x) 1 Γ(x)Γ(1 − x)= = B(x, 1 − x)= sx−1(1 − s)−xds. Γ(1) 0 u Z Moreover, if we change the variable s = u+1 , the integral becomes 1 ∞ ux−1 u −x du sx−1(1 − s)−xds = 1 − (u + 1)x−1 u +1 (u + 1)2 Z0 Z0 ∞ ux−1 = du. 1+ u Z0 1.3.1. Lemma. For y ∈ (0, 1) we have ∞ u−y π du = . 1+ u sin πy Z0 4 D. MILICIˇ C´ Proof. We cut the complex plane along the positive real axis. On this region we − z y −y define the function z 7−→ 1+z with the argument of z equal to 0 on the upper side of the cut. This function has a first order pole at z = −1 with the residue e−πiy. CR -1 Cε If we integrate this function along a path which goes along the upper side of the cut from ǫ > 0 to R, then along the circle CR of radius R centered at the origin, then along the lower side of the cut from R to ǫ and finally around the origin along the circle Cǫ of radius ǫ, by the residue theorem we get R u−y z−y R u−y z−y du + dz − e−2πiy du − dz =2πie−πiy. 1+ u 1+ z 1+ u 1+ z Zǫ ZCR Zǫ ZCǫ First we remark that for z 6= 0 we have |z−y| = |e−y log z| = e−y Re(log z) = e−y log |z| = |z|−y. Hence, the integrand in the second and fourth integral satisfies z−y |z|−y |z|−y ≤ ≤ . 1+ z |1+ z| |1 − |z|| Hence, for small ǫ we have z−y ǫ1−y dz ≤ 2π ; 1+ z (1 − ǫ) ZCǫ and for large R we have z−y R1−y dz ≤ 2π ; 1+ z (R − 1) ZCR Clearly, this implies that → 0 as R → ∞ and → 0 as ǫ → 0. This implies CR Cǫ that R ∞ u−y R 1 − e−2πiy du =2πie−πiy. 1+ u Z0 NOTESONRIEMANN’SZETAFUNCTION 5 It follows that ∞ u−y eπiy − e−πiy du =2πi 1+ u Z0 and finally ∞ u−y π du = . 0 1+ u sin πy Z This lemma implies that π π Γ(x)Γ(1 − x)= = . sin π(1 − x) sin πx for x ∈ (0, 1). Since both sides are meromorphic, it follows that the following result holds. 1.3.2. Proposition. For all z ∈ C, we have π Γ(z)Γ(1 − z)= . sin πz Since z 7−→ sin πz is an entire function, the right side obviously has no zeros. So, Γ(z) = 0 is possible only at points where z 7−→ Γ(1 − z) has poles. Since the poles of Γ are at 0, −1, −2, ··· , it follows that poles of z 7−→ Γ(1 − z) are at 1, 2, 3, ··· . At these points Γ(n +1)= n! 6= 0. Therefore, we proved the following result. 1.3.3. Theorem. The function Γ has no zeros. 2. Zeta function 2.1. Meromorphic continuation. Riemann’s zeta function ζ is defined by ∞ 1 ζ(z)= nz n=1 X for Re z > 1. In that region the series converges uniformly on compact sets and represents a holomorphic function. If we consider the expression for the Gamma function ∞ Γ(z)= tz−1e−tdt Z0 for Re z > 0, and change the variable t into t = ns for n ∈ N, we get ∞ Γ(z)= nz sz−1e−nsds, Z0 i.e., we have Γ(z) ∞ = tz−1e−ntdt nz Z0 for any n ∈ N and Re z > 0. This implies that, for Re z > 1, we have ∞ ∞ Γ(z)ζ(z)= tz−1e−ntdt n=1 0 X Z ∞ ∞ ∞ e−t ∞ tz−1 = tz−1 e−nt dt = tz−1 dt = dt. 1 − e−t et − 1 0 n=1 ! 0 0 Z X Z Z This establishes the following integral representation for the zeta function. 6 D. MILICIˇ C´ 2.1.1. Lemma. For Re z > 1 we have ∞ tz−1 Γ(z)ζ(z)= dt. et − 1 Z0 This integral can be split in to two parts ∞ tz−1 1 tz−1 ∞ tz−1 Γ(z)ζ(z)= dt = dt + dt.
Details
-
File Typepdf
-
Upload Time-
-
Content LanguagesEnglish
-
Upload UserAnonymous/Not logged-in
-
File Pages12 Page
-
File Size-