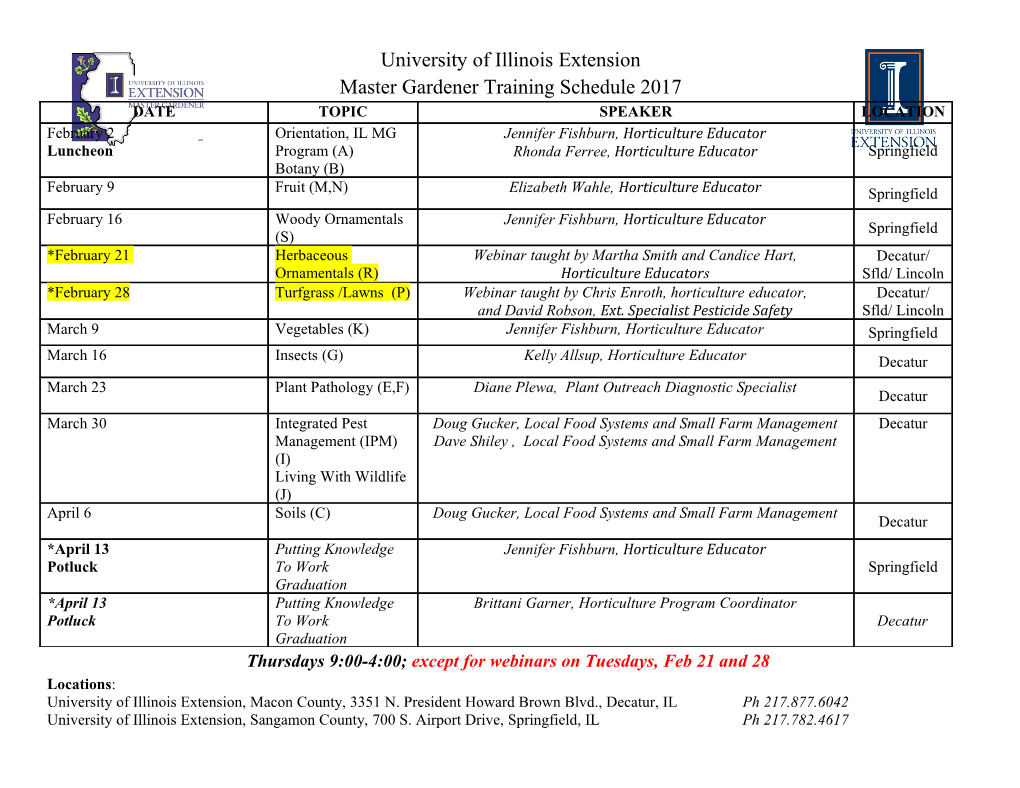
APPENDIX A STATE OF THE ART REVIEW A.1 Hydraulic and Geomorphic Background A wide variety of low-flow in-stream structures have been used to promote channel stability and prevent bank erosion that could threaten transportation infrastructure. Many of these structures are designed to reduce lateral river migration, especially in highly sinuous bends, and therefore reduce risks to infrastructure located on the floodplain. Other structures attempt to provide grade control within a stream at equilibrium. Note, however, that any of these structures will eventually fail in an actively degrading system, which is therefore not considered further here. In general these structures are not effective at reducing scour associated with in-stream transportation infrastructure, including bridge piers and abutments, since they have minimal effect on flow during flood events and therefore limited impact on scour prevention during these events. In fact, these structures may actually induce unintended scouring, especially when construction has not been completed properly (Dahle, 2008). Scour is, however, a major threat to the long-term stability of low-flow in-stream structures. The term scour denotes the removal of bed material caused by high shear stresses at the bed created by induced pressure gradients, energetic coherent vortices, and turbulence fluctuations. The scour directly attributed to an obstruction within the flow is defined as local scour (Melville and Coleman, 2000; Lagasse et al., 2001a; TAC, 2004). Scour around bridge piers, abutments, and exposed foundations has been studied extensively in the literature (Richardson and Davis, 2001). Even though bridge-foundation scour is not the focus of the current work, the information gained from these studies is highly relevant, as it can assist us in understanding the stability of in-stream structures. Lateral migration is also a consideration when evaluating the total scour at a flow obstruction, including an in-stream structure. Lateral migration can affect the total scour by changing the flow angle of attack (Richardson and Davis, 2001). Three specific flow features lead to local scour around a flow obstruction. A pressure gradient on the upstream face of the obstruction causes downflow which impinges upon bed material at the base. A highly dynamic large-scale vortical structure is created by this action which transports dislodged particles away from the obstruction; this eddy is referred to as a horseshoe vortex (Lagasse et al., 2001a). Additionally, wake vortices caused by separation as flow passes the obstruction entrain sediments through suction (Melville and Coleman, 2000; Richardson and Davis, 2001; TAC, 2004). Figure A-1 shows vortices and scour holes created by a vertical cylinder within the flow obtained from recent numerical simulations using SAFL’s VSL. Obstructions such as in-stream structures that reduce the available river width have the effect of constricting the flow at these points. Even the presence of a single rock or concrete obstruction will inevitably constrict the flow. This constriction creates an area of higher velocity and in turn, higher shear stress which leads to contraction scour. In periods of high flow, the effect is even more pronounced and the severity of scour increases accordingly. Contraction scour and local scour can ultimately compromise the stability of the structure itself (Richardson and Davis, 2001; TAC, 2004). For example, Figure A-2 shows a bridge that has undergone dangerous scour levels as a result of the local and contraction scour induced by the foundation. A-1 Figure A-1. Turbulent flow patterns in the vicinity of a vertical cylinder obtained from high- resolution numerical simulations. Left: Instantaneous vortical structures showing the complexity of the turbulent horseshoe vortex; Right: Scour hole and bed forms induced by the vortices colored with instantaneous bed shear stress (Escauriaza 2008; Escauriaza and Sotiropoulos 2008). Figure A-2. Photograph of bridge at low flow revealing severe flood-induced scour. Color changes on the bridge pier indicate original bed elevation (Baldwin, 1997). The magnitude of scour depends on geomorphic factors such as climate and topography, sediment size, flooding frequency, and in-stream infrastructure geometry. The magnitude will also be influenced by the presence of scour countermeasures and their effectiveness (Lagasse et al., 2001b). Final scour depth is achieved when equilibrium between erosive capability and bed resistance to motion is reached (Melville and Coleman, 2000). A-2 Erosion is the displacement of soil particles due to wind or water action (Lagasse et al., 2001a). Erosion, like scour, is the result of fluid forces acting on sediment and causing it to be dislodged and transported. Flow patterns, especially those located within stream meanders, are often highly erosive. If banks are allowed to erode, infrastructure such as bridge abutments can become undermined and fail. Accelerated erosion, sometimes referred to as mass wasting, is normally the result of high flows (Lagasse et al., 2001a). Erosive forces during high flows can be 100 times greater than during normal or low-flow periods. Additionally, 90% of all changes to a river occur during these infrequent periods of high flow (Richardson et al., 2001). Groundwater levels, bank material, stratification, and freeze-thaw cycles all play a role in determining the erosion potential of a channel (Simons, 1995). In addition, erosion can threaten the long-term stability of in-stream flow control structures. For example, Figure A-3 shows that severe bank erosion can undermine and expose bank-mounted infrastructure, here a bridge abutment. In addition, flow control structures will often alter bank erosion adjacent to and downstream from the structure. Figure A-3. Abutment scour caused by April 1979 flood, Leake County, MS (Colson, 1979). Bank erosion has been observed to be especially pronounced at the apex of meander bends due to pressure-driven secondary currents. These are helicoidal flow patterns, directed from the outer to the inner bank along the channel bed, that result from the local imbalance between the curvature-induced transverse pressure gradient and the centrifugal force along the bed. Secondary currents result in the increase of the water surface elevation on the outside bank, which is known as super-elevation, and redistribute streamwise momentum within the channel cross-section. Pressure-driven secondary circulation can persist downstream leading to undesired scour or depositions (ACOE, 1991). In both meandering and straight reaches, unchecked scour at the toe of banks will eventually lead to their failure (Odgaard, 1988). Channel stability can be determined by the use of geomorphic, hydraulic, and analytical stability A-3 assessments. Field reconnaissance must be completed to determine basic information about the channel and watershed it flows through (Rosgen, 2006). A theoretical model can then be used to asses channel stability. An appropriate software model that combines several field indicators of the reach stability with the desired final hydraulic geometry can be utilized to create a stable channel design (Copeland et al., 2001). A.2 Submerged Vanes Introduction This section summarizes available information on submerged vanes. Submerged vanes, sometimes called Iowa vanes, are thin foils angled into the upstream flow and submerged even during low flow periods. They are stream training structures designed to mitigate stream bank erosion and rebuild bed elevation by inducing secondary circulation. This action stabilizes the stream and prevents it from moving laterally. Submerged vanes are normally used in arrays which counter erosive secondary circulations triggered by channel meanders and are generally not in contact with the bank (Odgaard and Wang, 1991a). An examination of the underlying theory as well as laboratory work and case studies is presented here. This thorough review of literature suggests that submerged vanes are a promising stream stabilizing technique and should be studied in further detail. The cost of submerged vane projects has been found to be less, in most cases, than comparable bank armoring techniques (i.e. riprap; Keown et al., 1981) and no reported cases of extensive maintenance have been published. Submerged vanes are useful in a variety of applications ranging from the aforementioned prevention of bank erosion and lateral migration to the protection of bridge piers, abutments, and intake structures. Submerged vanes can also have the added benefit of being an aesthetically pleasing solution; when designed correctly, sediment and vegetation tend to bury the vanes leaving the stream in a more natural looking state. There are currently, however, only a handful of cases where submerged vane use has been documented in the field. As a result, their effectiveness in many settings is unknown as are their ecological impacts. Theory Submerged vanes were developed to prevent bank erosion and failure in curved channel sections. It is believed that the first study of vane applications in flow training was developed by Russian engineers Potapov and Pyshkin (1947). The first theoretical design works are credited to Odgaard and Kennedy (1983). They concluded that vanes are to be arranged in such a manner as to induce a secondary current which counteracts motion triggered by stream sinuosity and topography. The fluid motion caused by stream sinuosity is the end result of centrifugal forces which are present
Details
-
File Typepdf
-
Upload Time-
-
Content LanguagesEnglish
-
Upload UserAnonymous/Not logged-in
-
File Pages170 Page
-
File Size-