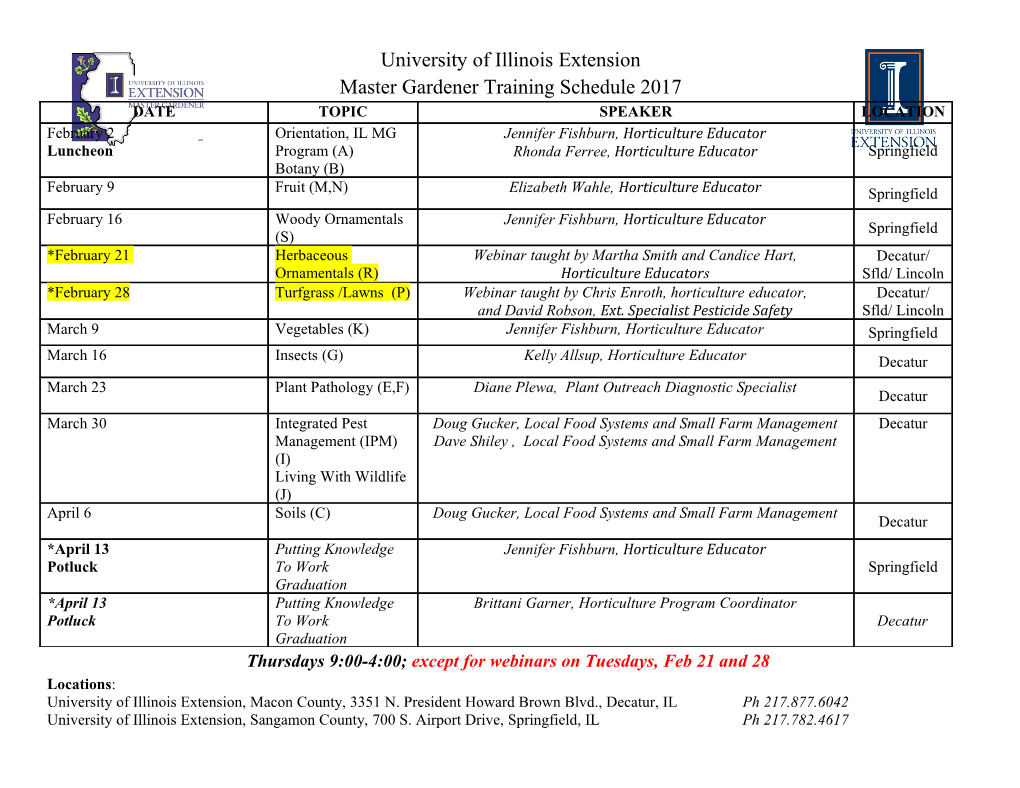
Current Status of r-Process Nucleosynthesis T. Kajinoa,b,c, W. Aokia, A. B. Balantekind, R. Diehle,f, M.A. Famianog, G.J. Mathewsh aNational Astronomical Observatory of Japan, Mitaka, Tokyo, Japan bUniversity of Tokyo, Tokyo, Japan cBeihang University, Beijing, China dUniversity of Wisconsin, Madison 53706 U.S.A. eMax Planck Institut für Extraterrestrische Physik, Garching, Germany fTechnical University of Munich, Germany gWestern Michigan University, U.S.A. hUniversity of Notre Dame, U.S.A. Abstract The rapid neutron capture process (r-process) is believed to be responsible for about half of the production of the elements heavier than iron and contributes to abundances of some lighter nuclides as well. A uni- versal pattern of r-process element abundances is observed in some metal-poor stars of the Galactic halo. This suggests that a well-regulated combination of astrophysical conditions and nuclear physics conspires to produce such a universal abundance pattern. The search for the astrophysical site for r-process nucle- osynthesis has stimulated interdisciplinary research for more than six decades. There is currently much enthusiasm surrounding evidence for r-process nucleosynthesis in binary neutron star mergers in the multi- wavelength follow-up observations of kilonova/gravitational-wave GRB170807A/GW170817. Nevertheless, there remain questions as to the contribution over the history of the Galaxy to the current solar-system r-process abundances from other sites such as neutrino-driven winds or magnetohydrodynamical ejection of material from core-collapse supernovae. In this review we highlight some current issues surrounding the nuclear physics input, astronomical observations, galactic chemical evolution, and theoretical simulations of r process astrophysical environments with goal of outlining a path toward resolving the remaining mysteries of the r-process. arXiv:1906.05002v1 [astro-ph.HE] 12 Jun 2019 Email addresses: [email protected] (T. Kajino), [email protected] (W. Aoki), [email protected] (A. B. Balantekin), [email protected] (R. Diehl), [email protected] (M.A. Famiano), [email protected] (G.J. Mathews) Preprint submitted to Elsevier June 13, 2019 Contents 1 Introduction 3 2 Nuclear Theory 6 2.1 Theoretical Neutron Capture Rates . .6 2.2 Theoretical Nuclear Masses . .6 2.3 Theoretical Nuclear Structure . .7 2.4 Theoretical β-Decay Rates . .7 2.5 β-delayed neutron emission . .8 2.6 Fission Barriers and Fission Fragment Distribution . .8 2.7 Neutrino Physics . .9 3 Experimental Nuclear Data 10 3.1 Nuclear Masses . 11 3.1.1 Mass Measurement Compilations and Evaluations . 13 3.1.2 Trap Measurements . 13 3.1.3 TOF Measurements . 15 3.1.4 Storage Rings . 18 3.1.5 Other Similar Systems . 19 3.2 β-decay rates and spectroscopy . 20 3.2.1 RIKEN . 20 3.2.2 CERN ISOLDE . 21 3.2.3 ANL/CARIBU . 21 3.2.4 NSCL . 22 3.2.5 GSI . 22 3.2.6 Jyväskylä . 23 3.2.7 Other Facilities . 23 3.2.8 Charge Exchange . 23 3.3 β-delayed neutron emission . 24 3.4 Fission Barriers and Distributions . 24 3.5 β-delayed fission . 25 3.6 Neutron Capture Rates . 26 3.6.1 n\TOF . 26 3.6.2 GSI-LAND . 26 3.6.3 Other Facilities and Techniques . 26 3.7 Nuclear Structure Studies . 27 3.7.1 Neutron Separation Energies and Shell Closures . 27 3.7.2 Isomers . 27 3.8 Current and Future Facilities . 27 3.8.1 RIBF . 27 3.8.2 FRIB . 28 3.8.3 RAON . 28 3.8.4 GSI-FAIR . 28 4 Candidate Astrophysical Environments 28 4.1 The Basics . 29 2 5 Astronomical Observations 30 5.1 Messengers of the r Process . 30 5.2 The Solar Abundance Reference . 32 5.3 Observing an r-Process Event . 34 5.3.1 Gamma Rays from Radioactivities . 34 5.3.2 Kilonova Emission and GW170817 . 35 5.4 Metal-poor Stars in our Galaxy . 35 5.4.1 The Abundance Pattern in the Stellar Archeological Record . 39 5.4.2 Abundance Pattern Variabilities . 39 5.4.3 Light Neutron-Capture Elements . 40 5.5 Stellar Abundances in other Galaxies . 40 5.6 Abundances of Interstellar Gas in the Galaxy . 42 5.6.1 r-Process Material on Earth . 42 5.6.2 Constraints from Pre-solar Grains . 45 5.7 Astronomical Summary on the r Process . 46 6 Sites for the r Process 46 6.1 Neutrino Driven Winds . 46 6.2 Magnetic Neutrino-Driven Wind . 47 6.3 r-process in low-mass prompt supernova explosions . 47 6.4 Neutron Star Mergers . 47 6.5 Black hole-Neutron Star Mergers . 48 6.6 Magneto-hydrodynamic Jet Models . 49 6.7 I-process . 50 6.8 Collapsar r Process . 50 6.9 r-process from dark matter induced black hole collapse . 51 6.10 The tr-Process . 51 7 Summary and Prospects 52 1. Introduction The origin of most atomic nuclei with masses above that of iron group elements (as well as some lighter elements) is attributed to neutron capture nucleosynthesis. Two different categories for neutron capture have been identified, depending upon the competition between neutron capture and β decay: If neutron capture rates are slow compared to β decay rates, only isotopes near stability are synthesized. This has been termed the slow-neutron capture process, s-process [1, 2]. On the other hand, when neutron captures occur much more rapidly than β-decay rates, isotopes far from stability are synthesized. This is called the rapid-neutron capture process, r-process [1], or fast [2] process. Successive and rapid neutron capture on a particular element can build up neutron-rich isotopes to the point at which photo-disintegration reaction rates equal those of neutron capture. At this point the (n; γ) (γ; n) equilibrium is maintained until the waiting-point isotope can β-decay to the next element. This process of neutron capture and β decay then repeats along a reaction path far from stability. This is called the r-process path. It is described in more detail in 6. From the effect of nuclear closed shells on the final r-process abundances, one can deduce that the r-process path runs far from the line of nuclear stability, so that very high neutron fluxes must occur. Moreover, because of the short β-decay times far from stability, the process only requires a short duration, typically tens of seconds or less to synthesize nuclei all the way from the iron group to actinide elements. One way of regulating the r process is via fission recycling. As neutron captures and β decays proceed to the heaviest nuclei, eventually the r process is terminated by neutron-induced or β-induced fission. The two fission fragments with intermediate-masses 120 . A . 160 can then continue to undergo neutron captures and β decays. This re-cycles the r process, and the repeated flow through the entire r-process path results in 3 a reduced dependence on the astrophysical environment, thus enhances the importance of nuclear properties for determining the isotopic abundances. The s-process, on the other hand, can occur with comparably low neutron fluxes inside stars. This process is generally associated [3] with episodic neutron irradiation of pre-existing nuclear matter in stars during the asymptotic giant branch stage of stellar evolution. The nuclear physics and astrophysics for s-process nucleosynthesis are more accessible both experimen- tally and theoretically than that for the isotopes relevant to the r process. Therefore, one typically evaluates the s-process nucleosynthesis in the solar system abundances and subtracts them from the total of the heavy- element abundances, to deduce the solar r-process abundance distribution; this is described below in 5. An important stimulus for studies of the r-process has been the elemental abundance pattern seen in spectroscopic studies of emission from the stellar-atmospheres of metal-poor stars in the Galactic halo. Such stars occasionally exhibit the same elemental r-process abundance pattern as known from the solar system abundances, This pattern appears to be rather universal even for stars at much younger ages and metallicities than the Sun. Even though the iron content, as a proxy for metallicity, varies over orders of magnitude, this elemental abundance pattern is rather robust for elements in the range of Z = 50–75 [e.g. 4, 5]. Thus, the enrichment from the r process appears to have occurred very early in the cosmic chemical evolution in the Galaxy. Moreover, it appears to to have operated the same way as it did to produce the solar abundances attributed to the r process. These observational facts suggest a rather well-regulated origin of heavy elements beyond iron. However, we do not know if this may be only an artifact of nuclear properties such as binding energies and β-decay rates, or it may point to a single cosmic site with astrophysical conditions that are generated uniformly throughout cosmic time. There appears to be a relatively large scatter of abundances at low metallicities compared to the light α-elements produced in supernovae. This indicates that such r-process nucleosynthesis events may be attributable to only one or very few events, and that these events are rather rare compared to occurrence rate of supernovae. The absolute abundances of r-process elements are about seven orders of magnitude below.
Details
-
File Typepdf
-
Upload Time-
-
Content LanguagesEnglish
-
Upload UserAnonymous/Not logged-in
-
File Pages72 Page
-
File Size-