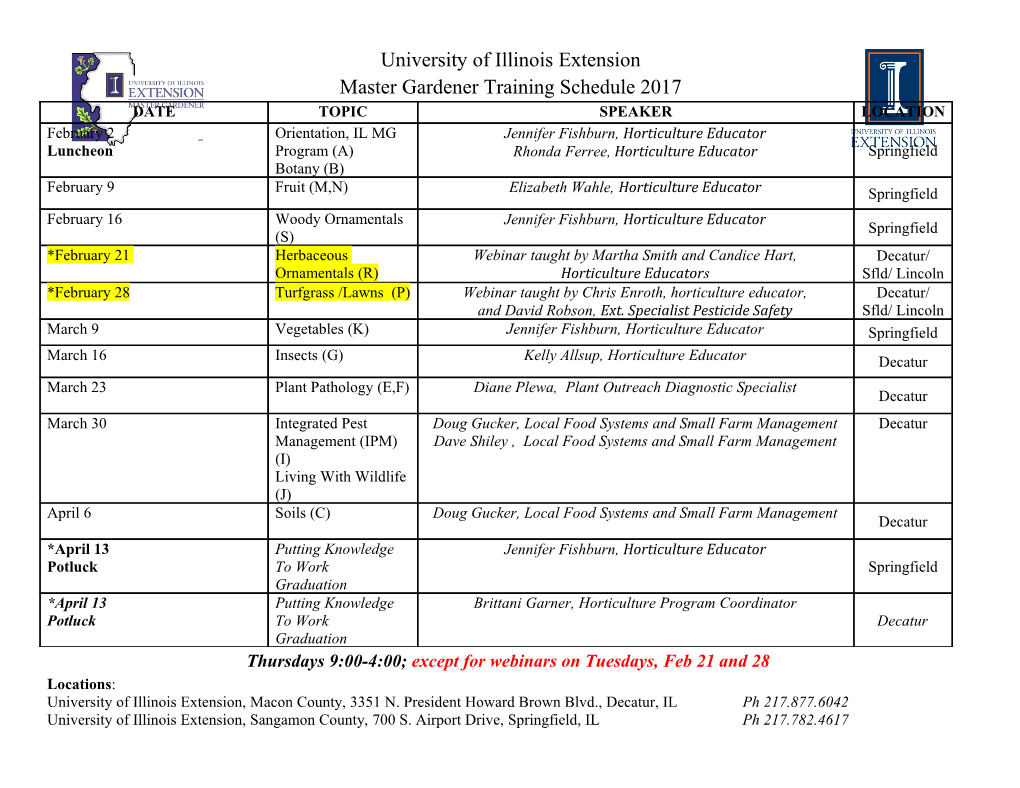
th Proceedings of the 5 International Workshop on Hydrogen and Fuel Cells October 26 –29, 2010 Campinas, SP, Brazil ISSN 2179-5029 SINTERING AND ELECTRICAL PROPERTIES OF STRONTIUM - DOPED LANTHANUM MANGANITE (1) DIEGO PEREIRA TARRAGÓ (2) BERTA MORENO BURRIEL (2) EVA CHINARRO MARTIN (2) JOSÉ RAMÓN JURADO EGEA (3) CÉLIA DE FRAGA MALFATTI (1) VÂNIA CALDAS DE SOUSA (1)LABIOMAT – PPGEM – UFRGS, Porto Alegre, RS, Brazil (2) CSIC – ICV, Madrid, Spain (3) LAPEC – PPGEM – UFRGS, Porto Alegre, RS, Brazil ABSTRACT Lanthanum strontium manganites (LSM) are potential materials for cathode applications in solid oxide fuel cells (SOFC) due to their good catalytic activity, chemical stability and compatibility with electrolyte materials in high temperatures. The sinterability of single phase La 1-xSr xMnO 3 (x=0.18) perovskite powders and the electrical properties of the resulting samples are analyzed in this study. Using a heating microscope, the powders were pressed and sintered at different pressures and temperatures, resulting in an open porosity of 33.36% when compacted at 125 MPa and sintered at 1200°C. Top and cross-section s canning electron microscopy (SEM) micrographs revealed interconnected pores in the sintered body and, hence, a suitable microstructure for the application. The activation energy for conductance was 0.04 eV and the tested LSM bulk started to exhibit adequate electrical properties at about 500°C. KEYWORDS : Perovskite; Lanthanum manganite; Solid oxide fuel cell; Cathode; Conductivity. 1. INTRODUCTION The development of devices such as solid oxide fuel cells (SOFC) requires the production of materials focusing on cost effectiveness rather than on the ideal properties for the application, while simultaneously reaching a compromise among the properties. The choice of cathode materials depends on parameters such as the electrolyte material, operating temperature and fuel cell design [1]. In this context, lanthanum strontium manganites (LSM) are promising materials for cathode applications when using yttria-stabilized zirconia (YSZ) as electrolyte material, especially due to the physical and chemical compatibility of these materials at high operating temperatures [2]. A reliable electrical characterization of LSM requires the preparation of a sintered bulk to avoid obtaining results influenced by poor crystallization, foreign ions, poor intergrain contact and residual stress [3]. In addition, the bulk’s open porosity must range from 30 to 40% and remain stable at operating temperatures [4]. 2. OBJECTIVES The aim of this work is to mold and sinter a LSM bulk with a microstructure suitable for cathode applications and to determine its electrical conductivity at different temperatures. 1 Correspondence should be addressed to Diego Pereira Tarragó: Phone: +55 (51) 3308-9485; e-mail: [email protected] 188 th Proceedings of the 5 International Workshop on Hydrogen and Fuel Cells October 26 –29, 2010 Campinas, SP, Brazil ISSN 2179-5029 3. METHODOLOGY LSM powders produced via combustion synthesis (La 1-xSr xMnO 3; x=0.18) with 8.7m²/g specific surface area and a mean particle diameter of 163 nm were initially tested in a heating microscope (Hess Instruments) from room temperature to 1300°C, applying a heating rate of 10°C/h. This test allowed for the identification of the sintering onset temperature and the maximum densification temperature. The LSM powders were then subjected to uniaxial pressures of 90, 125 and 160 MPa. To simplify the powder preparation process, the mold was lubricated only with glycerin, without any sintering additives. The samples where then sintered at temperatures ranging from 1150°C to 1300°C for 2 hours. The Archimedes method was used to determine the open porosity, and surface and cross section micrographs of the sintered bodies were recorded with a Jeol JSM-5800 scanning electron microscope. A slow scan X-ray diffraction pattern of a sintered body was generated in a Philips diffractometer (0.01°/5s) to confirm that t he tested bulk was a single phase LSM. Lastly, the d.c. electrical conductivity of the powders pressed into compacts at 125 MPa (18.39 mm diameter and 2.14 mm height) and sintered at 1200ºC was measured by a four-probe technique, according to the method proposed by Green and Gunn [5], using a multimeter (AGILENT 34401 A) and a power supply (Agilent E3646A), at temperatures varying from 25°C to 700°C. Pure platinum was used as the electrode. The electrical conductivity of the samples was calculated by Equation 3.1, where σ is the conductivity, s is the sample’s thickness and R is its resistance. ln 2 σ = (S ) Eq. 3.1 π.s.R cm The activation energy for the electronic conductivity was calculated graphically based on the calculated electrical conductivity. 4. RESULTS The curve traced by the heating microscope (see Figure 1) was used to determine the following parameters for the sintering tests. 1,02 1 0,98 0,96 Shrinkage 0,94 0,92 0,9 300 400 500 600 700 800 900 1000 1100 1200 1300 Temperature (°C) Figure 1: Powder retraction as a function of temperature. 189 th Proceedings of the 5 International Workshop on Hydrogen and Fuel Cells October 26 –29, 2010 Campinas, SP, Brazil ISSN 2179-5029 Figure 2 shows the LSM sintering curve which indicates the best variables for the desired open porosity. A further characterization was carried out of the sample pressed at 125 MPa and sintered at 1200°C. Figure 3 shows SEM micrographs of the sample’s microstructure. 55 90MPa 125MPa 50 160MPa 45 40 35 OpenPorosity (%) 30 25 20 1125 1150 1175 1200 1225 1250 1275 1300 1325 Temperature (°C) Figure 2: LSM sintering curve from 1150°C to 1300°C . (a) (b) Figure 3: SEM micrographs of the sample pressed at 125 MPa and sintered at 1200°C, showing: (a) top and (b) cross section, under 9000 X magnification. Figure 4 depicts the X-ray diffraction pattern of the sintered body, showing only the peaks of LSM rhombohedral perovskite (ICDD 01-089-0648). The plot in Figure 5 shows the bulk’s conductivity at the tested temperatures. 190 th Proceedings of the 5 International Workshop on Hydrogen and Fuel Cells October 26 –29, 2010 Campinas, SP, Brazil ISSN 2179-5029 6000 5000 4000 3000 Counts 2000 1000 0 20 30 40 50 60 70 2 ө Figure 4: X-ray diffraction pattern of the sample pressed at 125 MPa and sintered at 1200°C. 300 250 200 150 100 Conductivity (S/cm) 50 0 0 100 200 300 400 500 600 700 Temperature (°C) Figure 5: Effect of temperature on the conductivity of the sample pressed at 125 MPa and sintered at 1200°C. Figure 6 shows a log( σ) plot vs. 1000/T. The calculated conductivity activation energy was 0.04 eV. 2,5 2 ) ) (S/cm) σ 1,5 Log ( Log 1 0,5 1 1,5 2 2,5 3 3,5 1000/T (K -1 ) Figure 6: Graphical plot of the activation energy for electrical conductivity. 191 th Proceedings of the 5 International Workshop on Hydrogen and Fuel Cells October 26 –29, 2010 Campinas, SP, Brazil ISSN 2179-5029 5. DISCUSSION The results obtained with the heating microscope revealed that sintering started at 1100°C, based on the beginning of retraction, which is consistent with preliminary results reported by Ghosh et al. [6]. The maximum densification rate occurred at 1235°C. Therefore, to allow for densification of the bulk, green bodies must be sintered above 1100°C and, probably, around the maximum densification rate to reach the desired porosity. This justifies the temperature range used in this study. The powders were initially tested at compaction pressures of 90 and 160 MPa, but the resulting porosity was either too high or too low, although it decreased consistently with rising temperature. Thus, an intermediate compaction pressure of 125 MPa was tested, which resulted in the desired porosity of 33.36%, followed by a sintering temperature of 1200°C. It should be noted that when LSM is processed together with YSZ electrolyte, chemical reactions at their interface take place above 1250°C, with po ssible dielectric phase segregation [7]. SEM micrographs of this sample showed a promising microstructure with a good pore distribution both at the surface and in the cross section, with apparently good pore connectivity. Such microstructure favors the rapid transport of reactants to the reactive cathode/electrolyte interface, enhancing the electrode’s performance [1]. After the heat treatment at 1200°C, the resulting samples for electric characterization remained single phase LSM perovskite, as indicated by the X-ray diffraction analysis, thus providing reliable results. Figure 5 shows LSM exhibiting typical semiconductor behavior, with conductivity increasing with rising temperatures, starting at low conductivity values of less than 20 S/cm. The LSM bulk showed high electrical conductivity above 300°C, re maining quite stable up to the temperatures employed in this study. Moreover, the bulk did not undergo semiconductive to metallic transition, which occurs above 800°C according to L i et al. [7]. At 500°C the electrical conductivity exceeded 200 S/cm, exhibiting the performance of a real SOFC cathode and comparable to the electrical conductivity of some MIEC (mixed ionic and electronic conductor) cathodes [8]. Conductivity at the maximum studied temperature (700°C) was about 250 S/cm, which is a promising result considering the small amount of dopant added to the perovskite (x=0.18). This result was attributed to the fact that at this temperature, such compositions usually show lower conductivity and larger concentrations of dopant are normally required to reach this level of performance [9]. 6. CONCLUSIONS The sintering study reported here demonstrated that LSM powders can be sintered without additives and at temperatures suitable for sintering in contact with the YSZ electrolyte.
Details
-
File Typepdf
-
Upload Time-
-
Content LanguagesEnglish
-
Upload UserAnonymous/Not logged-in
-
File Pages6 Page
-
File Size-