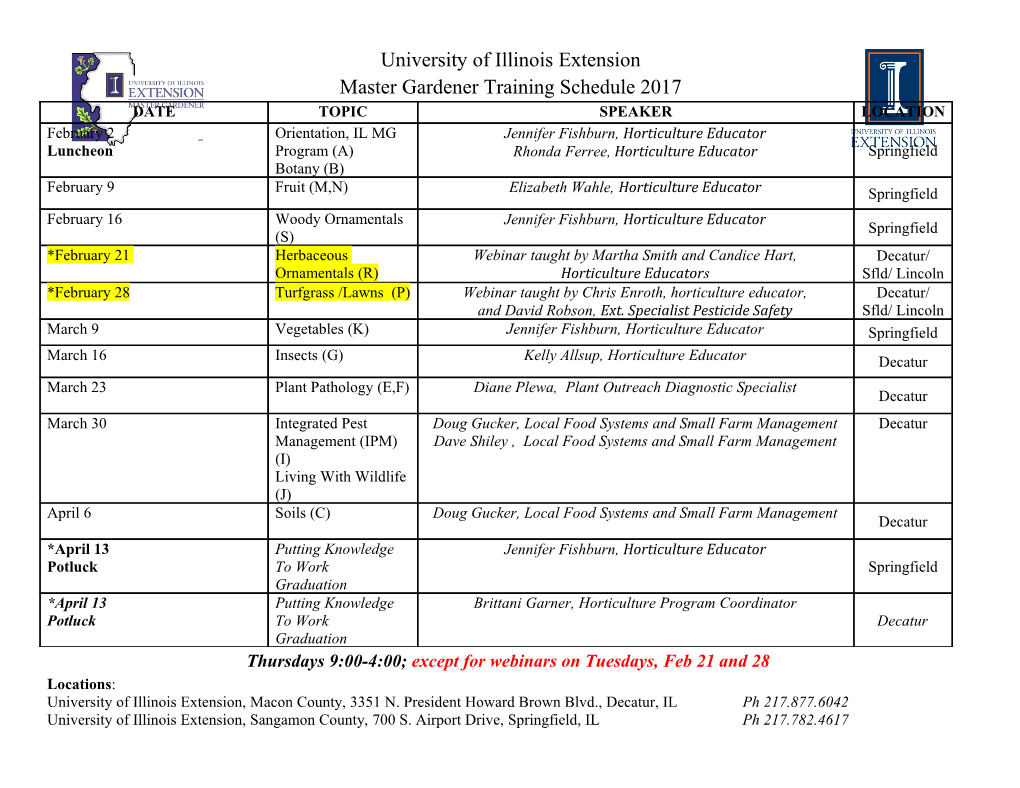
Chapter 10 Cholesterol-Sphingomyelin Interactions in Cells-Effects on Lipid Metabolism J. Peter Slotte 1. INTRODUCTION Both cholesterol and sphingomyelin are important constituents of cellular plasma membranes. The molecules are chemically and functionally very different, yet they appear to be attracted to each other in the membrane compartment. It is the aim of this review to discuss how alterations in their membrane interactions may affect lipid homeostasis in cells, and to suggest a molecular explanation for their mutual affinity in membranes. Recent reviews dealing with the subcellular distribution and transport of cholesterol (Liscum and Dahl, 1992; Liscum and Faust, 1994; Liscum and Under­ wood, 1995), with cellular lipid traffic (van Meer, 1989; Pagano, 1990; Voelker, 1991; Allan and Kallen, 1993), with transport and metabolism of sphingomyelin (Koval and Pagano, 1991), and with the role of sphingolipids in cell signaling (Kolesnick, 1991, 1994; Hannun and Bell, 1993; Hannun, 1994), may also be of interest to the reader. 2. CELLULAR DISTRIBUTION OF CHOLESTEROL 2.1. Cell Cholesterol Homeostasis Cholesterol is an essential component of cellular plasma membranes in higher organisms. Cholesterol interacts with membrane phospholipids and J. Peter SloUe Department of Biochemistry and Pharmacy, Abo Akademi University, FIN 20520 Turku, Finland. Subcellular Biochemistry, Volume 28: Cholesterol: Its Functions and Metabolism in Biology and Medi­ cine, edited by Robert Bittman. Plenum Press, New York. 1997. R. Bittman (ed.), Cholesterol 277 © Plenum Press, New York 1997 278 J. Peter Slotte influences their physico-chemical properties. The important membrane proper­ ties that are directly or indirectly influenced by membrane levels of cholesterol include solute permeability (for a review, see Yeagle, 1985), phospholipid acyl chain mobility (GaIly et ai., 1976; Stockton and Smith, 1976, Yeagle, 1985) and lateral packing (Chapman et ai., 1969; Lund-Katz et ai., 1988; Smaby et ai., 1994). Cholesterol also has a marked influence on lateral phase separations (Smutzer and Yeagle, 1985), and on the effective free volume of membranes (Straume and Litman, 1987), two parameters that are directly related to the flexi­ bility of membrane proteins (e.g., ion channels, enzymes) and hence to their func­ tion in membranes. Since cholesterol is such an essential and ubiquitous molecule in cells, most eucaryotic cells have retained the capacity to synthesize cholesterol from two­ carbon precursor molecules. An important and early rate-limiting step in the biosynthesis of cholesterol is the conversion of ~-hydroxy-~-methylglutaryl-CoA to mevalonate, catalyzed by ~-hydroxy-~-methylglutaryl-CoA reductase (HMG­ CoA reductase; Brown et ai., 1973). The regulation of HMG-CoA reductase in­ cludes (long-term) transcriptional regulation, probably by oxygenated sterols, as well as inactivation/activation of the enzyme by an AMP-dependent protein kinase/phosphatase cascade (Beg et aI., 1978; Ingebritsen et ai., 1981; Brown and Goldstein, 1986; Mehrabian et aI., 1986; Gibson and Parker, 1987). An alternative source of cellular cholesterol is provided by low density lipoproteins (LDL), which bind to plasma membrane apo BIE receptors (Goldstein and Brown, 1977). The receptorlLDL complex is internalized by a process termed receptor-mediated endocytosis (Brown and Goldstein, 1986), in which the recep­ tor ligand is transported to the lysosomal compartment for partial or complete degradation (Brown et aI., 1975). Unesterified cholesterol in the lysosomes (released from the LDL particles or derived from the hydrolysis of LDL-derived cholesteryl esters) is transported directly to the plasma membrane compartment. Eventually, any excess of plasma membrane unesterified cholesterol may be trans­ ported to the endoplasmic reticulum for conversion to cholesteryl esters (Tabas et aI., 1988). Rates of LDL internalization and cellular cholesterol esterification (by acyl-CoA:cholesterol acyl transferase; ACAT) are sensitive to cellular levels of unesterified cholesterol (Goldstein and Brown, 1977). An unregulated pathway for cellular uptake of lipoprotein-derived unesterified cholesterol involves the di­ rect (surface) transfer of lipoprotein surface cholesterol to the cellular plasma membrane compartment. This pathway may contribute significantly to cell choles­ terol accumulation when the extracellular level ofLDL is high (Slotte et ai., 1988). Since extrahepatic and nonsteroidogenic cells cannot metabolize cholesterol, save for the conversion of cholesterol to cholesteryl esters, routes must exist whereby cells can rid themselves of excess cholesterol. High-density lipoproteins have been shown to act as cholesterol acceptors, and initiate the reverse-cholesterol transport system first proposed by Glomset in 1968 (Glomset, 1968; Rothblat and Phillips, 1991). It is at present unclear which of the HDL subtypes are the best cho- Cholesterol-Sphingomyelin Interactions 279 Ie sterol acceptor particles, although their capacities to initiate cholesterol efflux from cells clearly differ (Rothblat et aI., 1992; Mahlberg and Rothblat, 1992; Davidson et at., 1994). Whereas the actual transfer of cholesterol from cellular plasma membranes to extracellular acceptor particles apparently do not require binding proteins (Johnson et aI., 1991), the interaction of HDL-like particles with specific cell surface binding proteins may lead to a regulated flow (translocation) of intracellular cholesterol to the cell surface (Slotte et aI., 1987; Mendez et aI., 1991; Porn et at., 1991a). 2.2. Subcellular Distribution of Cholesterol Most of the cellular unesterified cholesterol can be found in the plasma mem­ brane compartment. Depending on the cell type examined and the assay method used, plasma membranes have been reported to contain from about 50% to as much as 90% of the total cellular unesterified cholesterol (Lange, 1991; Warnock et aI., 1993). It appears that cells with an extensive recycling of plasma membranes through the en­ docytic system have a larger fraction of intracellular cholesterol (Allan and Kallen, 1993). It is not surprising that cellular plasma membranes contain such a high con­ centration of cholesterol, since several of the cellular cholesterol transport pathways are directed toward the cell surface. Newly synthesized cholesterol is transported vec­ tori ally from the intracellular site of synthesis to the cell surface, where it appears with a transfer half-time of 10-18 min (DeGrella and Simoni, 1982; Lange et aI., 1991; Urbani and Simoni, 1990; Kaplan and Simoni, 1985). Exogenously derived choles­ terol, entering the cell with LDL particles, is also quantitatively transported from lyso­ somes to the cell surface, where it accumulates (Tabas et aI., 1988). Only when the solubilizing capacity of the plasma membrane compartment is saturated does the ex­ cess cholesterol move to the endoplasmic reticulum for esterification by ACAT (Xu and Tabas, 1991). It is now established that the capacity of plasma membranes to solu­ bilize cholesterol is largely a function of its sphingomyelin content (see section 5). 3. SYNTHESIS AND DISTRIBUTION OF SPHINGOMYELIN 3.1. Site of Sphingomyelin Synthesis in Cells Sphingomyelin appears to be synthesized by the enzymatic transfer of the phosphocholine moiety of phosphatidylcholine to ceramide (catalyzed by sphingo­ myelin synthase), thus yielding sphingomyelin and diacylglycerol (Voelker and Kennedy, 1982; Spence et at., 1983; Marggraf and Kanfer, 1984; Kishimoto, 1993). The direct transfer of CDP-choline to ceramide is apparently a pathway of minor significance in most cells (Voelker and Kennedy, 1982). There is currently a dispute regarding the major site of sphingomyelin synthesis, since synthetic activ­ ities have been demonstrated (directly or indirectly) in several different cellular 280 J. Peter Slotte compartments (Futerman et aI., 1990; van Echten et aI., 1990; leckel et aI., 1990; Kallen et aI., 1993, 1994). In studies using subcellular fractionation techniques (rat liver), it was observed that cis Golgi fractions accounted for the largest activity of sphingomyelin synthesis, whereas only a small fraction of the synthetic activity could be attributed to the plasma membrane fraction (Futerman et aI., 1990; leckel et aI., 1990). In baby hamster kidney cells, however, it was reported that a sub­ stantial synthesis of sphingomyelin occurred in a compartment distinct from the cis/medial Golgi. This compartment was presumed to include the endocytic recy­ cling pathway (Kallen et al., 1993, 1994). There is always the possibility that the predominant site of synthesis may differ from one cell type to another, as a differ­ ent synthetic topology may be needed for functionally specialized cells. This is in­ deed discussed in a recent report, where it was shown that the plasma and myelin membrane compartment accounted for at least half of the sphingomyelin synthase activity in oligodendrocytes (Vos et aI., 1995). 3.2. Subcellular Distribution of Sphingomyelin The distribution of sphingomyelin mass among intracellular organelles has been examined using cell fractionation studies and sphingomyelinase degradation experiments. Results with both techniques suggest that more than half of the cel­ lular sphingomyelin mass is confined to the plasma membranes (with most of it being in the exoleaflet; for a review, see Koval and Pagano, 1991). With fibro­ blasts it was reported that as much as 90% of the total cell sphingomyelin mass was in the plasma
Details
-
File Typepdf
-
Upload Time-
-
Content LanguagesEnglish
-
Upload UserAnonymous/Not logged-in
-
File Pages17 Page
-
File Size-