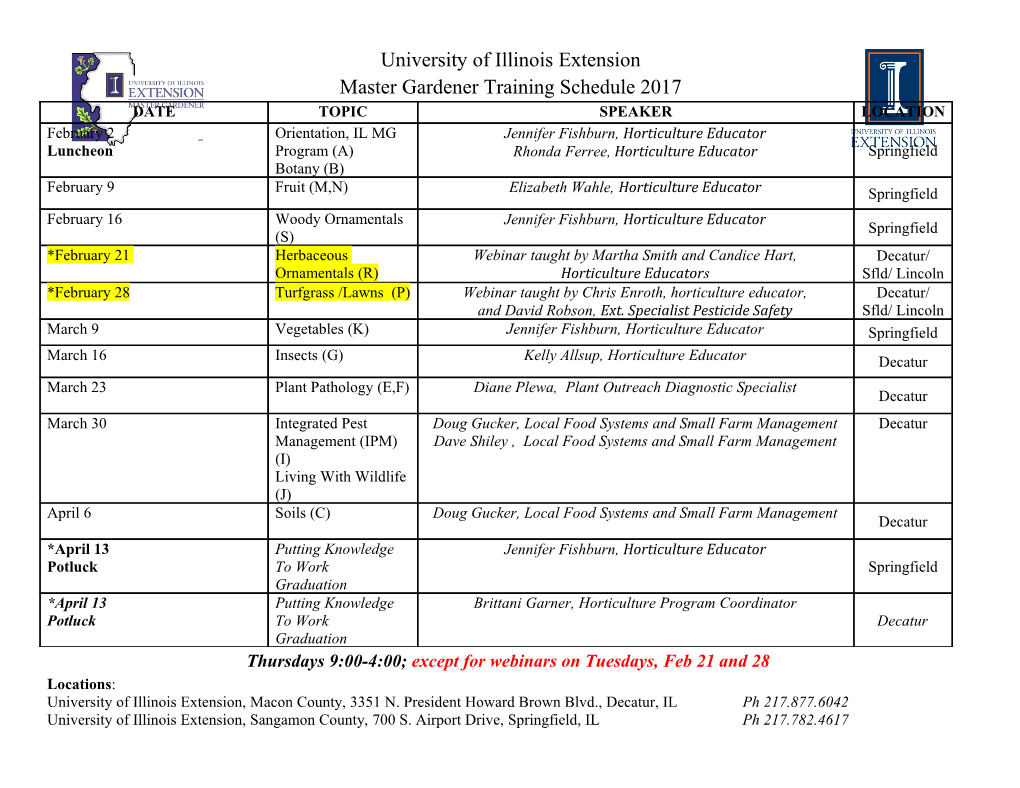
Supplement: The story behind meldonium–From pharmacology to performance enhancement: A narrative review Pharmacology and biochemical actions of L-carnitine and meldonium: The design of meldonium was aimed at interference with L-carnitine metabolism. L-carnitine, a quaternary amine, is a water-soluble molecule that is especially important in mammalian metabolism for the mitochondrial oxidation of fatty acids. Meldonium acts as a carrier for the transport of activated long-chain fatty acids from the cytosol into the mitochondria, where - oxidation and ATP synthesis take place. The balance of L-carnitine is maintained through biosynthesis, kidney reabsorption, and nutrition intake, particularly from red meat and milk. [1,2] About 25% of L-carnitine in the human organism is derived by endogenous biosynthesis from the amino acids lysine and methionine in the liver and kidney. More than 99% of the body’s L- carnitine can be found intracellularly, mainly in the liver, heart, and skeletal muscle. Because L- carnitine cannot be degraded or metabolised, it needs to be eliminated, mainly in the urine. In the pathway of carnitine biosynthesis, enzymatic processes performed by mitochondrial trimethyllysine aldolase (TMLD), 3-hydroxy-trimethyllysine aldolase (HTMLA) and trimethylaminobutyrate dehydrogenase (TMABA) by the formation of -butyrobetaine (GBB) play a central role. In the final step, GBB is hydroxylated by GBB hydroxylase (BBOX) in order to form L-carnitine.[3] The transport of L-carnitine through cell membranes is performed via Na+ -dependent organic cation transporters (OCTN), mainly OCTN2 and to a lesser extent OCTN1 (figure to supplement). After the activation of fatty acids with a CoA group, the acyl-CoA units are converted into carnitine esters, catalysed by the carnitine palmytoiltransferase I (CPT I), resulting in long-chain acylcarnitine esters. The CPT I-mediated L-carnitine transport is one of the rate-limiting steps of free fatty-acid oxidation, because low carnitine concentrations can inhibit free fatty-acid transport to mitochondria.[4,5] These esters are transported over the inner mitochondrial membrane by carnitine-acylcarnitine translocase (CACT) and are further transesterified to intramitochondrial CoA on the mitochondrial matrix side by carnitine palmitoyltransferase II (CPT II). Acyl-CoA is formed, and these long-chain CoA complexes enter -oxidation, resulting in the formation of acetyl-CoA needed for the citric acid cycle. Catalysed by carnitine acetyltransferase (CAT), the released L-carnitine forms complexes with acetyl residues, which can then leave the mitochondria via CACT. In addition, L-carnitine is involved in the recycling of CoA, facilitating the shuttling of short-chain acyl groups from the mitochondria to the cytosol (peroxisomes), which results in an increase of mitochondrial free CoA and thus better availability for further energy production.[6] L-Carnitine is also involved in the regulation of glucose metabolism via the modulation of the free coenzyme A/acetyl-coenzyme A ratio.[7] The main target of meldonium is the enzyme GBB hydroxylase, which can be inhibited competitively as well non-competitively.[8,9] The following main actions of meldonium have been reported (for a review, see [10-12] and figure to supplement). In humans, meldonium decreased the concentrations of L-carnitine and increased the plasma concentration of GBB.[13] The inhibition of -oxidation induced an increase in fatty-acid levels in the sera of treated rats. Meldonium blocked the transport of carnitine inside the mitochondria by a weak inhibition of CAT [14] and inhibited Na+ -dependent carnitine transport and CPT I activity. In addition, weak competitive inhibition of CACT was reported.[15] Meldonium increased the protein content of hexokinase type 1 and sarcoplasmic reticulum Ca2+-ATPase, activated the reuptake of Ca2+ by the sarcoplasmic reticulum, [16-18] and improved the uptake of Ca2+ ions in the sarcoplasmic reticulum.[19] In addition, it induced an increase in renal L-carnitine excretion [13] and a competitive inhibition of OCNT2 proteins by the renal brush-border membrane.[9] In total, the consequences of meldonium applications are: a) a shift of cell metabolism from highly oxygen- consuming fatty-acid oxidation to increased glucose consumption and increased effectiveness of ATP generation and b) a protection of the mitochondria against free fatty-acid overload by the reduction of long-chain acylcarnitines, the activation of mitochondrial free fatty-acid utilization, and the redirection of fatty-acid metabolism from the mitochondria to the peroxisomes. Legend to Figures: Figure to Supplement: Cellular transport of L-carnitine and fatty acids and mitochondrial energy-metabolism pathways including the sites of meldonium actions (adapted from [4, 19]). Abbreviations: inhibition by meldonium; activation by meldonium; OMM, outer mitochondrial membrane; IMM, inner mitochondrial membrane; OCTN2, organic cation/carnitine transporter 2; CPT, carnitine palmytoil-transferase; CACT, carnitine acyl-carnitine translocase; LC-Acyl-CoA, long-chain acyl-coenzyme A; CoASH, free coenzyme A; glucose-6-P, glucose-6- phosphate; PDC, pyruvate dehydrogenase complex; LC-acylcarnitine, long-chain acylcarnitine; LC-acyl-CoA, long-chain acyl-coenzyme A; CAT, carnitine acetyl-transferase Reference List 1. Rebouche CJ. Kinetics, pharmacokinetics, and regulation of L-carnitine and acetyl-L-carnitine metabolism. Ann N Y Acad Sci 2004;1033:30-41 2. El-Hattab AW, Scaglia F. Disorders of carnitine biosynthesis and transport. Mol Genet Metab 2015;116(3):107-112 3. Strijbis K, Vaz FM, Distel B. Enzymology of the carnitine biosynthesis pathway. IUBMB Life 2010;62(5):357-362 4. Vaz FM, Wanders RJ. Carnitine biosynthesis in mammals. Biochem J 2002;361(Pt 3):417-429 5. Pochini L, Scalise M, Galluccio M, et al. OCTN cation transporters in health and disease: role as drug targets and assay development. J Biomol Screen 2013;18(8):851-867 6. Wanders RJ, Vreken P, Ferdinandusse S, et al. Peroxisomal fatty acid alpha- and beta- oxidation in humans: enzymology, peroxisomal metabolite transporters and peroxisomal diseases. Biochem Soc Trans 2001;29(Pt 2):250-267 7. Stephens FB, Constantin-Teodosiu D, Greenhaff PL. New insights concerning the role of carnitine in the regulation of fuel metabolism in skeletal muscle. J Physiol 2007;581(Pt 2):431-444 8. Simkhovich BZ, Shutenko ZV, Meirena DV, et al. 3-(2,2,2-Trimethylhydrazinium)propionate (THP)--a novel gamma-butyrobetaine hydroxylase inhibitor with cardioprotective properties. Biochem Pharmacol 1988;37(2):195-202 9. Spaniol M, Brooks H, Auer L, et al. Development and characterization of an animal model of carnitine deficiency. Eur J Biochem 2001;268(6):1876-1887 10. Sjakste N, Gutcaits A, Kalvinsh I. Mildronate: an antiischemic drug for neurological indications. CNS Drug Rev 2005;11(2):151-168 11. Sjakste N, Kalvinsh I. Mildronate: An antiischemic drug with multiple indications. Pharmacologyonline 2006;1:1-18 12. Dambrova M, Makrecka-Kuka M, Vilskersts R, et al. Pharmacological effects of meldonium: Biochemical mechanisms and biomarkers of cardiometabolic activity. Pharmacol Res 2016 13. Liepinsh E, Konrade I, Skapare E, et al. Mildronate treatment alters gamma-butyrobetaine and l-carnitine concentrations in healthy volunteers. J Pharm Pharmacol 2011;63(9):1195-1201 14. Jaudzems K, Kuka J, Gutsaits A, et al. Inhibition of carnitine acetyltransferase by mildronate, a regulator of energy metabolism. J Enzyme Inhib Med Chem 2009;24(6):1269-1275 15. Oppedisano F, Fanello D, Calvani M, et al. Interaction of mildronate with the mitochondrial carnitine/acylcarnitine transport protein. J Biochem Mol Toxicol 2008;22(1):8-14 16. Hayashi Y, Ishida H, Hoshiai M, et al. MET-88, a gamma-butyrobetaine hydroxylase inhibitor, improves cardiac SR Ca2+ uptake activity in rats with congestive heart failure following myocardial infarction. Mol Cell Biochem 2000;209(1-2):39-46 17. Heidemanis K, Balcere I, I k. Mildronate-plasma membrane interaction mechanisms. Proc Latv Acad Sci 1990;11:108-115 18. Yonekura K, Eto Y, Yokoyama I, et al. Inhibition of carnitine synthesis modulates protein contents of the cardiac sarcoplasmic reticulum Ca2+-ATPase and hexokinase type I in rat hearts with myocardial infarction. Basic Res Cardiol 2000;95(5):343-348 19. Hayashi Y, Muranaka Y, Kirimoto T, et al. Effects of MET-88, a gamma-butyrobetaine hydroxylase inhibitor, on tissue carnitine and lipid levels in rats. Biol Pharm Bull 2000;23(6):770-773 .
Details
-
File Typepdf
-
Upload Time-
-
Content LanguagesEnglish
-
Upload UserAnonymous/Not logged-in
-
File Pages5 Page
-
File Size-