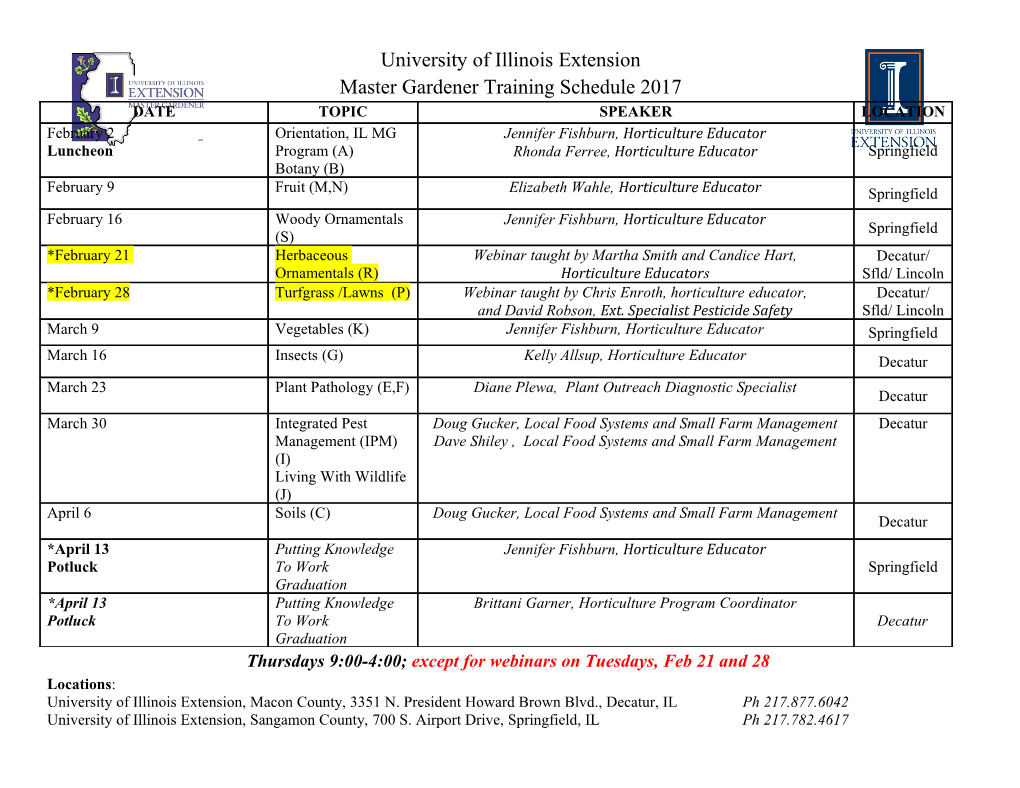
C. T.-C. Nguyen, “High-Q micromechanical oscillators and filters for communications,” Proceedings of the 1997 IEEE International Symposium on Circuits and Systems, Hong Kong, June 9-12, 1997, pp. 2825-2828. High-Q Micromechanical Oscillators and Filters for Communications Clark T.-C. Nguyen Center for Integrated Sensors and Circuits Department of Electrical Engineering and Computer Science University of Michigan 2406 EECS Bldg., 1301 Beal Ave., Ann Arbor, Michigan 48109-2122, email: [email protected] ABSTRACT W With Q’s in the tens to hundreds of thousands, microma- h chined vibrating resonators are proposed as IC-compatible MEMS Transducer tanks for use in the low phase noise oscillators and highly Resonator Capacitor L selective filters of communications systems. To date, LF Electrode oscillators have been fully integrated using merged 2 µm CMOS+microstructure technologies, and bandpass filters SAW Resonator Resonator consisting of coupled resonators have been demonstrated in Beam the HF range. The performance of fabricated two- and three- Quartz Interdigital Anchor Substrate Transducers Silicon resonator filters with center frequencies ranging from 1000X 300kHz to 10 MHz and filter Q’s from 100 to 2400 are Magnification Die reported. Evidence suggests that the ultimate frequency range of this high-Q tank technology depends upon material limita- 1 cm tions, as well as design constraints—in particular, to the degree of electromechanical coupling achievable in micro- scale resonators. 1 cm 1 cm Fig. 1: Size comparison between present-day SAW resonator I. INTRODUCTION technology and the described high-Q µmechanical The majority of the high-Q bandpass filters commonly resonator technology. used in the RF and IF stages of heterodyning transceivers are realized using off-chip, mechanically-resonant components, of present efforts aimed at both size reduction and perfor- such as crystal filters and SAW devices. Due to higher quality mance enhancement of transceivers via miniaturization of factor Q, such technologies greatly outperform comparable high-Q signal processing elements. Specific results will be filters implemented using transistor technologies, in insertion reported, including a review of integrated oscillator work and loss, percent bandwidth, and achievable rejection [2]. High Q of recently demonstrated micromechanical resonators and fil- is further required to implement local oscillators or synchro- ters in the HF range. The remainder of this paper then nizing clocks in transceivers, both of which must satisfy strict focuses upon projections for the ultimate frequency range phase noise specifications. Again, off-chip elements (e.g., and performance of these communications devices. quartz crystals) are utilized for this purpose. Being off-chip II. ADVANTAGES OF MEMS components, the above mechanical devices must interface with integrated electronics at the board level, and this consti- Reduced size constitutes the most obvious incentive for tutes an important bottleneck to miniaturization and perfor- replacing SAW’s and ceramics by equivalent µmechanical mance of heterodyning transceivers. For this reason, recent devices. The substantial size difference between microme- attempts to achieve single-chip transceivers for paging and chanical resonators and their macroscopic counterparts is cellular communications have utilized direct conversion illustrated in Fig. 1, which compares a typical SAW resona- architectures, rather than heterodyning, and have suffered in tor with a clamped-clamped beam micromechanical resona- overall performance as a result [1]. In this respect, single- tor [7] of comparable frequency. The particular µresonator chip solutions to heterodyning transceivers are desirable. shown is excited electrostatically via parallel-plate capacitive The rapid growth of micromachining technologies, transducers and designed to vibrate in a direction parallel to which yield high-Q on-chip vibrating mechanical resonators the substrate with a frequency determined by material prop- [3], may now make miniaturized, single-chip heterodyning erties, geometric dimensions, and stress in the material. Typi- transceivers possible. With Q’s of over 80,000 [4] under vac- cal dimensions for a 100 MHz micromechanical resonator uum and center frequency temperature coefficients in the are L≈12.9 µm, W=2 µm, and h=2 µm. With electrodes and 2 range of -10 ppm/oC (several times less with nulling tech- anchors, this device occupies an area of 420 µm = 0.00042 2 2 niques) [5], polycrystalline silicon micromechanical resona- mm . Compared with the several mm required for a typical tors (abbreviated “µresonators”) can serve well as VHF range SAW resonator, this represents several orders of miniaturized substitutes for crystals in a variety of high-Q magnitude in size reduction. oscillator and filtering applications. To date, LF (i.e., 20 kHz) A related incentive for the use of micromechanics is oscillators [6] and two-resonator prototypes of bandpass fil- integrability: Micromechanical structures can be fabricated ters in the HF range have been demonstrated [7,11,12]. For using the same planar process technologies used to manufac- use in cellular communications, however, much higher fre- ture integrated circuits. Several technologies demonstrating quencies must be achieved. This paper presents an overview the merging of CMOS with surface micromachining have 1 of 4 Sustaining y Electrode Resonator Amplifier Buffer Anchor R (Input) x Wr amp Comb-Transducer V R P vi Q1 L12 Shuttle V∆f Output Mass Lr 12 Electrode vi W C i i Folded-Beam P1 x2 R o R f Suspension Input h Q2 Electrode vo + Coupling CP2 Spring x Anchors z VP L Cx1 C′ C′ y − x1 12 12 Lx2 Rx2 R Co2 x1 Cx2 Co1 Fig. 2: SEM of a 16.5 kHz CMOS µresonator oscillator with C12 schematics explicitly depicting circuit topology. The µresonator occupies 420 × 230 µm2 Fig. 3: Perspective view schematic of the two-resonator µmechanical filter, along with the preferred bias, emerged in recent years [6,8,9], and one of these is now used excitation, and sensing circuitry, and the equivalent for high volume production of commercial accelerometers circuit for the filter. [8]. Using similar technologies, complete systems containing integrated micromechanical filters and oscillator tanks, as ∂C ∂x i = V ---------o ----- (1) well as sustaining and amplification electronics, all on a sin- o P ∂x ∂t gle chip, are possible. This in turn makes possible high-per- formance, single-chip transceivers, with heterodyning is then generated through the output transducer, which is then architectures and all the communication link advantages amplified by the transresistance sustaining amplifier and associated with them. Other advantages inherent with inte- directed back to the input comb-transducer, completing the gration are also obtained, such as elimination of board-level positive feedback loop required for oscillation. The transcon- µ parasitics that could otherwise limit filter rejections and dis- ductance behavior of the mechanical resonator can be mod- tort their passbands. eled by an LCR equivalent circuit [6,7] when designing this oscillator. III. MEMS COMPONENTS FOR TRANSCEIVERS The total area consumed by the oscillator of Fig. 2 is 420 The front-end of a wireless transceiver typically contains × 330 µm2. The measured phase noise floor of this oscillator a good number of off-chip, high-Q components that are is consistent with that of high-Q, communications-grade potentially replaceable by micromechanical versions. Among oscillators [6]. the components targeted for replacement are RF filters, Micromechanical Filters. including image rejection filters, with center frequencies Figure 3 presents the perspective view of a two-resona- ranging from 800 MHz to 2.5 GHz; IF filters, with center fre- tor, HF micromechanical filter, along with the preferred bias, quencies ranging from 455 kHz to 254 MHz; and high-Q, excitation, and sensing circuitry. As shown, the filter consists low phase noise oscillators, with frequency requirements in of two µmechanical clamped-clamped beam resonators, cou- the 10 MHz to 2.5 GHz range. pled mechanically by a soft spring, all suspended 0.1 µm Micromechanical Resonator Oscillators. above the substrate. Conductive (polysilicon) strips underlie The scanning electron micrograph (SEM) for a 16.5 kHz the central regions of each resonator and serve as capacitive micromechanical resonator oscillator, fully integrated with transducer electrodes positioned to induce resonator vibra- sustaining CMOS electronics, is shown in Fig. 2 [6]. To max- tion in a direction perpendicular to the substrate. The resona- imize frequency stability [10], a folded-beam, comb-trans- tor-to-electrode gaps are determined by the thickness of a duced micromechanical resonator is utilized [3]. As shown, sacrificial oxide spacer during fabrication and can thus be this µresonator consists of a finger-supporting shuttle mass made quite small (e.g., 0.1 µm or less), to maximize electro- suspended 2 µm above the substrate by folded flexures, mechanical coupling. which are anchored to the substrate at two central points. The Under normal operation, the device is excited capaci- shuttle mass is free to move in the x-direction indicated, par- tively by a signal voltage applied to the input electrode. The allel to the plane of the silicon substrate, with a fundamental output is taken at the other end of the structure, also via resonance frequency determined largely by material proper- capacitive transduction. Upon
Details
-
File Typepdf
-
Upload Time-
-
Content LanguagesEnglish
-
Upload UserAnonymous/Not logged-in
-
File Pages4 Page
-
File Size-