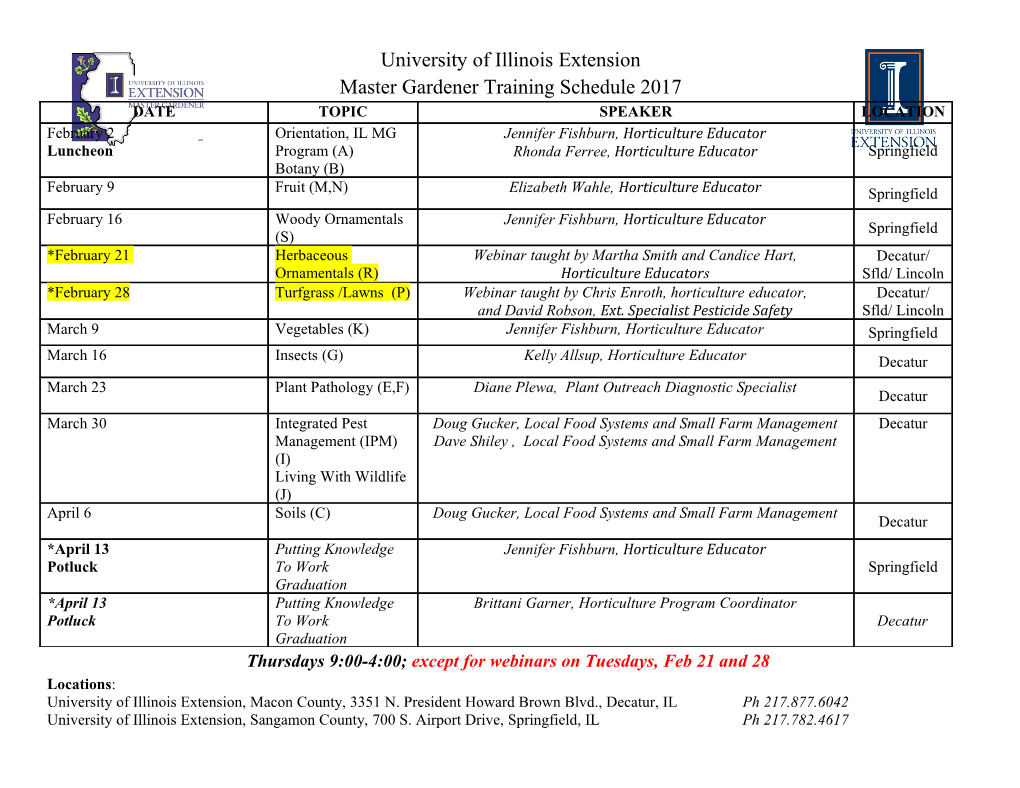
AdS/CFT Correspondence GEORGE SIOPSIS Department of Physics and Astronomy The University of Tennessee Knoxville, TN 37996-1200 U.S.A. e-mail: [email protected] Notes by James Kettner Fall 2010 ii Contents 1 Path Integral 1 1.1 Quantum Mechanics ........................ 1 1.2 Statistical Mechanics ........................ 3 1.3 Thermodynamics .......................... 4 1.3.1 Canonical ensemble . 4 1.3.2 Microcanonical ensemble . 4 1.4 Field Theory ............................. 4 2 Schwarzschild Black Hole 7 3 Reissner-Nordstrom¨ Black Holes 11 3.1 The holes . 11 3.2 Extremal limit . 13 3.3 Generalizations . 15 3.3.1 Multi-center solution . 15 3.3.2 Arbitrary dimension . 16 3.3.3 Kaluza-Klein reduction . 17 4 Black Branes from String Theory 19 5 Microscopic calculation of Entropy and Hawking Radiation 23 5.1 The hole . 23 5.2 Entropy . 25 5.3 Finite temperature . 27 5.4 Scattering and Hawking radiation . 29 iii iv CONTENTS LECTURE 1 Path Integral 1.1 Quantum Mechanics Set ~ = 1. Given a particle described by coordinates (q, t) with initial and final coor- dinates of (qi, ti) and (qf , tf ), the amplitude of the transition is ∗ hqi, ti | qf , tf i = hψ |qf , tf i = ψ (qf , tf ) . where ψ satisfies the Schrodinger¨ equation ∂ψ 1 ∂2ψ i = − . ∂t 2m ∂q2 Feynman introduced the path integral formalism Z −iS hqi, ti | qf , tf i = [dq] e q(ti)=qi,q(tf )=qf R where S = dtL is the action and L = L(q, q˙) is the Lagrangian, e.g., 1 L = mq˙2 − V (q) 2 We convert to imaginary time via the Wick rotation τ = it so 1 L → − mq˙2 − V (q) ≡ −L 2 E where the derivative is with respect to τ, LE is the total energy (which is bounded Rbelow), and the subscript E stands for Euclidean. The action becomes iS → SE = dτLE so the path integral becomes Z [dq] e−SE . 2 LECTURE 1. PATH INTEGRAL The major contribution to the path integral’s value comes from minimum SE, i.e., δS = 0 i.e., from the classical trajectory q = qcl(τ). Consider a perturbation of the classical trajectory q(τ) = qcl (τ) + δq (τ) S = S + ... Z cl Z [dq] e−SE = e−Scl [dq] e− ... −Scl hqi, ti | qf , tf i ≈ e The correlators are Z −SE hqi,τ i |T [q (τ 1) q (τ 2) ··· ]| qf,τ f i = [dq] q (τ 1) q (τ 2) ··· e q(τ i)=qi,q(τ f )=qf (1.1.1) where T denotes a time ordered product. Notice that the path integral automatically takes care of time ordering. This can easily be seen by splitting the path integral into time intervals bounded by τ 1, τ 2, ... We are interested in the vacuum expectation values G(τ 1, τ 2,... ) = h0 |T [q (τ 1) q (τ 2) ··· ]| 0i . (1.1.2) To write them in terms of a path integral, notice that by using the time evolution oper- ator, we have −τ f H |qf , τ f i = e |qf , 0i (1.1.3) where H is the Hamiltonian with eigenstatesP|ni; H |ni = En |ni and we take E0 = 0 (not necessary). Inserting the identity I = states |ni hn| in equation (1.1.3), X −τ f En |qf , τ f i = e |ni hn | qf , 0i states we see that in the limit τ f → ∞, all terms but one vanish. Therefore, |qf , τ f i → |0i h0 | qf , 0i Similarly, hqi, τ i| → hqi, 0 | 0i h0| as τ i → −∞. Substituting these limiting expres- sions into equation (1.1.1) gives R −SE h0 |T [q (τ 1) q (τ 2) ··· ]| 0i hqi, 0 | 0i h0 | qf , 0i = [dq] q (τ 1) q (τ 2) ... e R h0 | 0i hq, 0 | 0i h0 | q, 0i = [dq] e−SE R [dq]q(τ )q(τ ) ... e−SE G(τ , τ ,... ) = R 1 2 1 2 [dq]e−SE where we integrate over all trajectories q(τ) with τ ∈ (−∞, +∞) and no specified end points. 1.2 Statistical Mechanics 3 1.2 Statistical Mechanics Set the Boltzmann constant kB = 1. Denote temperature by T ≥ 0; At T = 0 the system settles in the ground state. Vacuum expectation values correspond to T = 0. To study finite T , introduce the partition function X Z = e−En/T states D ¯ ¯ E X ¯ ¯ = n ¯e−H/T ¯ n states X ³ ´ = Tr e−H/T |ni hn| states³ ´ = Tr e−H/T Z D ¯ ¯ E ¯ ¯ = dq q, 0 ¯e−H/T ¯ q, 0 Z = dq hq, 0 | q, 1/T i Z Z = dq [dq0] e−SE 0 0 Z q (0)=q (1/T )=q = [dq] e−SE q(0)=q(1/T ) Therefore, the partition function is given by a path integral over periodic orbits of period 1/T . 1 2 Example 1 Quantum mechanics: consider a free particle V = 0 and L = 2 mq˙ . Then using path integrals r m 2 −m(qf −qi) /2(τ f −τ i) hqi, ti | qf , tf i = e 2π(τ f − τ i) Example 2 Statistical mechanics: Set τ i = 0, τ f = 1/T , and qi = qf = q. Z r mT Z = dq which is infinite so put thesystem in a box 2π r mT = V where V is the volume (length). 2π which is the same as Z ∞ dp 2 Z = V e−p /2m 2π r−∞ mT = V . 2π 4 LECTURE 1. PATH INTEGRAL 1.3 Thermodynamics 1.3.1 Canonical ensemble Expand the partition function around the classical trajectory to get Z = e−Scl+ ... = e−F/T where F is the Helmholtz free energy so F = SclT. 1 −En/T The probability of a state |ni is pn = Z e . The internal energy is U = hEi X = pnEn states ∂ (ln Z) = T 2 ∂T The entropy is X ∂ (ln Z) U F S = p ln p = T − ln Z = − n n ∂T T T states which means F = U − TS. 1.3.2 Microcanonical ensemble The microcanonical ensemble is isolated from its environment. The number of states with a given energy E is given by the multiplicity g(E) = eS(E), i.e., the entropy counts the number of different states. X Z = e−E/T g(E) energy levels −E0/T ≈ g(E0)e = eS(E0)−E0/T ∂S 1 where E0 maximizes S(E) − E/T . We deduce ∂E = T . 1.4 Field Theory µ Set the speed of light c = 1. Let x = (t, x) be the positionR 4-vector. Consider a real 4 field ϕ(x) with dynamics governed by the actionR S = d xL where L (∂µϕ, ϕ) is the Lagrangian density; the Lagrangian is L = d3xL. Use the Wick rotation τ = it so 1.4 Field Theory 5 2 2 2 2 2 2 ds = −dt + dx = dτ + dx = dsE (Euclidean continuation). The correlation functions or Green functions are G(x , x ,... ) = h0 |T (ϕ(x )ϕ(x ) ··· )| 0i 1 2 R 1 2 [dϕ] ϕ (x ) ϕ (x ) ··· e−SE = R 1 2 [dϕ] e−SE as before. Taking an arbitrary function J as a source current, the generating functional is given by Z ³ ´ R 4 Z [J] = [dϕ] e−SE + d xJϕ and ¯ ¯ δ δ ¯ G(x1, x2.... ) = ··· Z [J] ¯ (1.4.1) δJ(x1) δJ(x2) J=0 Example 3 For a free field of mass m, 1 1 L = (∂ φ)2 + m2φ2 + Jφ E 2 µ 2 The classical field equation is the Klein-Gordon equation 2 2 −∇ φcl + m φcl = J Writing a quantum field as φ = φcl + δφ we obtain Z (2) 4 SE = Scl + S (δφ) ,Scl = d LE(∂clµ, φcl) Notice that there is no linear terms, because it is proportional to the field equation. Then Z[J] = e−Scl where we omitted a constant which was independent of J. We have Z 1 S = d4xd4x0J(x)D (x, x0)J(x0) cl 2 E where DE is the propagator, 2 2 0 4 0 (−∇ + m )DE(x, x ) = δ (x − x ) Using (1.4.1), we easily deduce G(x1, x2) = DE(x1, x2) You are invited to check that this is still valid when interactions are included. 6 LECTURE 1. PATH INTEGRAL LECTURE 2 Schwarzschild Black Hole Spacetime is provided with a metric tensor gµν so that a line element has length 2 µ ν ds = gµν dx dx 2 2 2 3 In flat spacetime, ds = −dt + dx (x ∈ R ), so gµν = ηµν = diag(−1 1 1 1) as a matrix. We denote the determinant of gµν by g. The Einstein equations are ½ 1 0 , with just gravity not matter R − Rg = . µν 2 µν source , in the presence of matter ν µ Rµν is the Ricci tensor (the contracted curvature tensor Rµνρ) and R = Rµ (its trace) 1 is the Ricci scalar. Rµν − 2 Rgµν = 0 arises from the action Z 1 √ S = d4x −gR. 16πG If we take the trace of the Einstein equation in empty space, we get 1 Rµ − Rgµ = 0 µ 2 µ which implies R = 0 so Rµν = 0. Schwarzschild found the solution dr2 ds2 = −f(r)dt2 + + r2dΩ2 f(r) 2 2 2 2 2 2 where dΩ2 = dθ + sin θdϕ is the line element on the sphere S and 2GM f(r) = 1 − r Where do G and M come from? Compare to electromagnetism with Maxwell’s equations without currents µν ∂µF = 0. 8 LECTURE 2: Schwarzschild Black Hole 2 Q Its simplest symmetric solution is Aµ = (A0, 0) with ∇ A0 = 0 so A = r , i.e.
Details
-
File Typepdf
-
Upload Time-
-
Content LanguagesEnglish
-
Upload UserAnonymous/Not logged-in
-
File Pages35 Page
-
File Size-