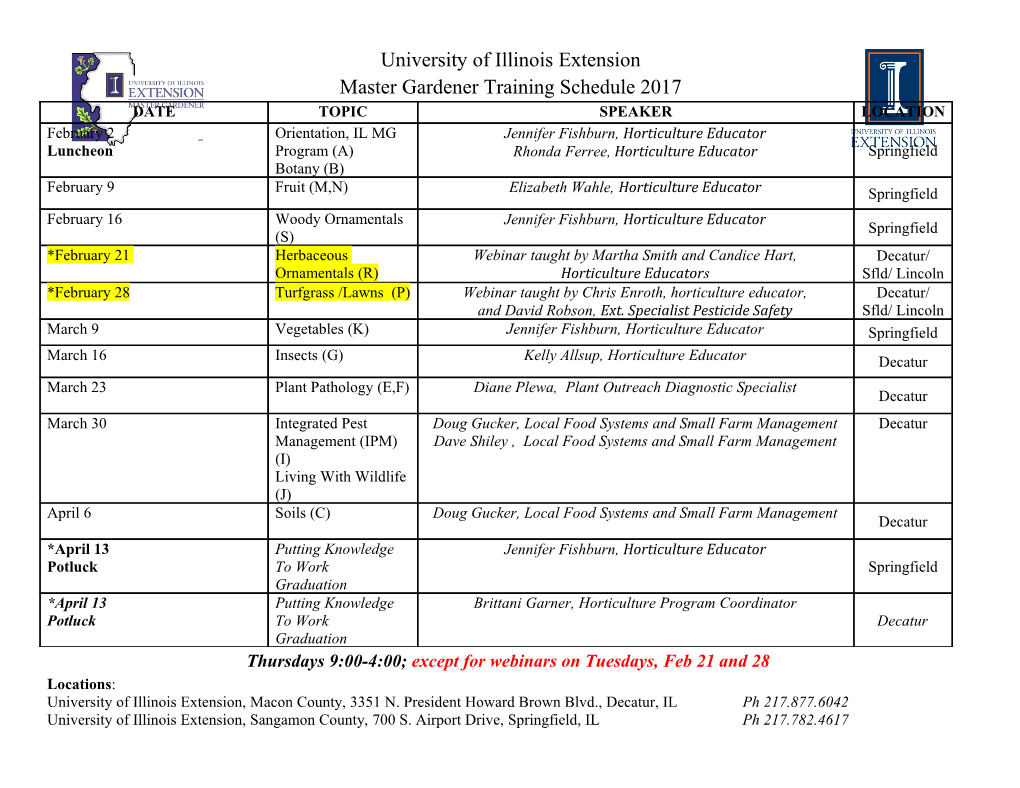
BIOCHEMICAL CHARACTERIZATION OF N-TERMINALLY TAGGED STYRENE MONOOXYGENASE FROM PSEUDOMONAS A thesis submitted to the faculty of San Francisco State University AS In partial fulfillment of The Requirements for The Degree Master of Science In • O U Chemistry: Biochemistry by Nonye Nwa-Niaf Okonkwo San Francisco, California May 2015 Copyright by Nonye N. Okonkwo 2015 CERTIFICATION OF APPROVAL I certify that I have read The Biochemical Characterization of N-Terminally Histidine Tagged Styrene Monooxygenase from Pseudomonas by Nonye Nwa-Niaf Okonkwo, and that in my opinion this work meets the criteria for approving a thesis submitted in partial fulfillment of the requirements for the degree: Master of Science in Chemistry: Biochemistry at San Francisco State University. Professor of Biochemistry Weiming Wu, PhD Professor of Biochemistry « BIOCHEMICAL CHARACTERIZATION OF N-TERMINALLY TAGGED STYRENE MONOOXYGENASE FROM PSEUDOMONAS Nonye Nwa-Niaf Okonkwo San Francisco State, California 2015 Flavoprotein monooxygenases are involved in a wide variety of biological oxidations due to their ability to utilize organic flavin cofactors as substrates to stereo- and regioslectively produce valuable bioactive compounds. Metabolism of styrene by Pseudomonas putida (S12) bacteria is accomplished by a two-component flavoenzyme system, which consists of styrene monooxygenase A (SMOA) and styrene monooxygenase B (SMOB), a reductase. Our present research evaluates the catalytic mechanisms of an N-terminally histidine-tagged version of the styrene monooxygenase reductase (NSMOB) component. Fluorescence monitored titrations at 4°C confirmed the equilibrium dissociation constant of NSMOB to have a Kd value of ~ 50 nM, an order of magnitude greater than the wild-type reductase. Steady-state kinetic analysis at 30°C also determined that a double-displacement mechanism with NADH as the leading substrate is the preferred method of FAD reduction. Due to the significant change in FAD binding affinity and catalytic mechanism, stopped-flow fluorescence and absorbance was used to evaluate the pre-steady state kinetics of NSMOB. We determined that the hydride-transfer rate constant is k = 48 s'1, which is identical to the wild-type enzyme at k = 50 s'1 at 15°C, which effectively resolves the rate-limiting step for the N-terminally tagged enzyme. These findings will be presented together with their implications for the engineering of N-terminally tagged enzymes and N-terminally linked fusion proteins as biocatalysts for the production of essential chiral epoxides. I certifWhat the Abstract is a correct representation of the content of this thesis. Date PREFACE AND AKNOWLEDGMENTS I would like to thank Dr. George Gassner for four years of exceptional mentorship and outstanding research experience. I would like to recognize Berhanegerbial Assefa for his preliminary work on the N-terminally tagged SMOB. Additionally, I would like to recognize the San Francisco State Women’s Association, College of Science and Engineering, PG&E, CSU Sally Casanova Pre-Doctoral Program and Undergraduate NIH-MARC and Graduate NIH-RISE MBRS fellowships for their continued financial support and professional development assistance. This work was supported by NIFISC1 GM081140 to George Gassner and Nonye Okonkwo was supported by the NIH-MARC 5T34-GM008574 and NIH- MBRS RISE fellowships. v TABLE OF CONTENTS List of Tables......................................................................................................................viii List of Figures.......................................................................................................................ix List of Equations.................................................................................................................. xi List of Appendices.............................................................................................................. xii Introduction.......................................................................................................................... 1 1.1 Significance and toxicology of styrene in the environment..........................1 1.2 Microbiology of styrene degradation.............................................................5 1.3 Styrene catabolic and detoxification pathway..............................................11 1.4 One- and two-component styrene monooxygenases.................................... 14 1.5 Catalytic mechanisms of styrene monooxygenases......................................18 1.6 Styrene monooxygenase structure.................................................................25 1.7 Naturally-occurring and artificially engineered fusion proteins................ 27 1.8 Putative complexes..........................................................................................30 1.9 Transition to thesis research............................................................................32 Methods............................................................................................................................... 34 2.1 Protein expression and purification...............................................................34 2.2 Activity and purity assays..............................................................................36 2.3 Time-dependent activity studies.................................................................... 39 2.4 Steady-state kinetic analysis ofNSMOB.....................................................40 2.5 Estimation of equilibrium dissociation constants........................................ 43 2.6 Pre-steady-state kinetics.................................................................................44 Results................................................................................................................................. 46 3.1 Flavin-refolded SMOB experiments with FAD, FMN, and riboflavin....46 3.2 Flavin-refolded SMOB time dependence studies........................................ 52 3.3 NSMOB mechanistic studies..........................................................................56 3.4 Estimation of equilibrium dissociation constants by fluorescence Monitored Titrations..............................................................................................60 vi 3.5 NSMOB single-turnover studies................................................................... 61 3.6 Efficiency of oxidative- and reductive half-reactions.................................65 Discussion........................................................................ 68 4.1 N-terminal tags and implications in protein engineering............................ 68 4.2 Isoalloxazine ring environment: SMOB refolding and stability................ 69 4.3 SMO Rate-limiting hydride-transfer step ....................................................72 4.4 SMO Flavin-transfer mechanisms and protein-protein interactions..........75 4.5 Engineered Flavin Monooxygenases as Efficient Biocatalysts.................77 References........................................................................................................................... 78 Appendix............................................................................................................................. 84 LIST OF TABLES Table Page 1. Flavin-refolded SMOB total protein and specific activity............................................... 50 2. Comparison of NSMOB Kinetic Parameters..................................................................... 57 3. Hull Hypothesis Comparison of BiBi Sequential and Double-Displacement Mechanisms............................................................................................................................... 59 LIST OF FIGURES Figure Page 1. Chemical Structure of Styrene and (S)-Styrene-7, 8- oxide........................................... 4 2. Metabolic fate of styrene.................................................................................................... 7 3. Bacterial degradation pathway for styrene....................................................................... 9 4. Styrene monooxygenase catalysis....................................................................................12 5. C4a-hydroperoxyflavin intermediate.............................................................................14 6. Oxidative and reductive half-reactions...........................................................................18 7. Isoalloxazine catalytic cycle of flavin-dependent monooxygenases........................... 23 8. N-terminally tagged SMOB.............................................................................................25 9. Overall structure of N-terminally tagged styrene monooxygenase A ......................... 26 10. Organization and mechanism of SMO systems........................................................... 28 11. Possible complex formation of native and N-terminally tagged SMOB and SMOA during the FAD-exchange reaction..................................................................................... 32 12. Flavin-refolding process of SMOB in 8M urea............................................................ 46 13. Flavin structure: riboflavin, FMN and FAD..................................................................47 14. Flavin-refolded SMOB SDS-PAGE..............................................................................49 15. Graph of flavin-refolded SMOB total protein assay results.......................................50 16.
Details
-
File Typepdf
-
Upload Time-
-
Content LanguagesEnglish
-
Upload UserAnonymous/Not logged-in
-
File Pages102 Page
-
File Size-