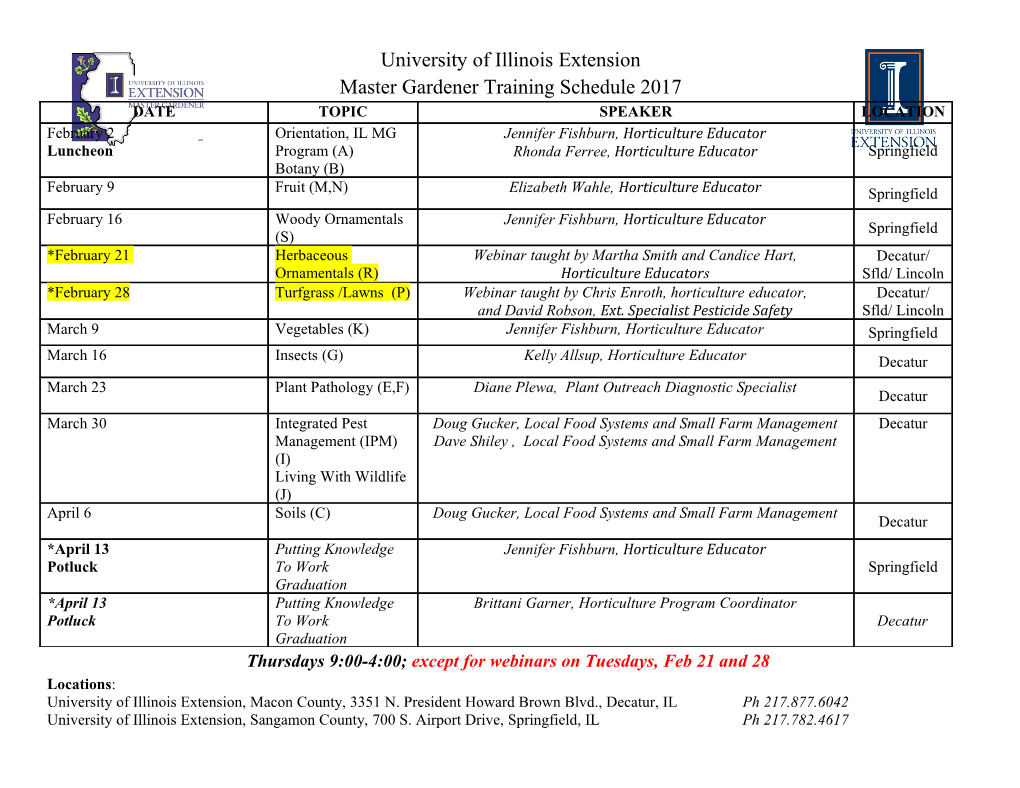
X-ray crystal structures elucidate the nucleotidyl transfer reaction of transcript initiation using two nucleotides Michael L. Gleghorna,1, Elena K. Davydovab,2, Ritwika Basua, Lucia B. Rothman-Denesb,3, and Katsuhiko S. Murakamia,3 aDepartment of Biochemistry and Molecular Biology, Pennsylvania State University, University Park, PA 16802; and bDepartment of Molecular Genetics and Cell Biology, University of Chicago, Chicago, IL 60637 Edited* by E. Peter Geiduschek, University of California at San Diego, La Jolla, CA, and approved December 30, 2010 (received for review November 6, 2010) We have determined the X-ray crystal structures of the pre- and in the structure and misalignment of the reactive groups of sub- postcatalytic forms of the initiation complex of bacteriophage N4 strate. In the present study, we have used X-ray crystallography RNA polymerase that provide the complete set of atomic images and a natural substrate plus a proper substrate analog to capture depicting the process of transcript initiation by a single-subunit a set of atomic resolution snapshots, from nucleotide binding RNA polymerase. As observed during T7 RNA polymerase transcript to nucleotidyl transfer reaction, (Fig. 1A and Table S1) to eluci- elongation, substrate loading for the initiation process also drives a date a complete picture of the process of transcript initiation conformational change of the O helix, but only the correct base by the central domain of N4 phage virion-encapsulated RNAP pairing between the þ2 substrate and DNA base is able to com- (mini-vRNAP). plete the O-helix conformational transition. Substrate binding also facilitates catalytic metal binding that leads to alignment of the re- Results active groups of substrates for the nucleotidyl transfer reaction. Design of the X-Ray Crystallographic Experiment to Monitor the For- Although all nucleic acid polymerases use two divalent metals for mations of Transcript Initiation Complexes. Previously, we reported catalysis, they differ in the requirements and the timing of binding the X-ray crystal structure of the binary complex (BC) of promo- of each metal. In the case of bacteriophage RNA polymerase, ter DNA and N4 mini-vRNAP (9), which is a member of the BIOCHEMISTRY we propose that catalytic metal binding is the last step before the T7-like single-subunit RNAP family (10) that recognizes a speci- nucleotidyl transfer reaction. fic DNA hairpin sequence with a 5-bp stem, 3-nt loop as its promoter (Fig. 1B) (11–13). In the BC structure, from −1 to þ2 NA-dependent RNA polymerases (RNAPs) transcribe DNA template DNA bases point toward the nucleotide entry pore, Dgenetic information into RNA and play a central role in gene whereas the þ3 template DNA base is flipped in the opposite expression. RNAP catalyzes a nucleotidyl transfer reaction, which direction providing an opportunity to analyze the structural tran- is initiated by the nucleophilic attack of an O3′ oxyanion at the sitions of DNA template bases at the þ1 and þ2 positions and RNA 3′ terminus to the α-phosphate (αP) of the incoming of the enzyme upon nucleotide loading. nucleotide, resulting in phosphodiester bond formation and re- The structures reported in this study represent the precatalytic lease of pyrophosphate (PPi). Both single-subunit T7 phage-like [substrate complex I (SCI); substrate complex II (SCII); mis- RNAPs and the multisubunit cellular RNAPs possess two nucleo- match complex (MC)] and postcatalytic [product complex (PC)] tide-binding sites for loading the RNA 3′ end (P site) and the stages of transcript initiation (Fig. 1A). Each complex comprises incoming NTP (N site) (1, 2). A two metal-ion catalytic mechan- the 120 kDa N4 mini-vRNAP and a 36-nt DNA, which includes ism has been proposed, as the enzyme possesses two divalent the P2 promoter 7 bp stem, stable and well-ordered 3-nt loop catalytic and nucleotide-binding metal cations chelated by two or hairpin followed by five bases of single-stranded DNA including three conserved Asp residues (3). The catalytic metal is a Lewis the start site (þ1) (Fig. 1B). Promoter and template DNA regions ′ acid, coordinating the RNA 3 -OH lowering its pKa and facilitat- to þ3 ∼ 4 were well resolved in the crystal structures, but were ing the formation of the attacking oxyanion. The nucleotide-bind- completely disordered downstream. The P2_7a DNA sequence ing metal is coordinated by the triphosphate of the incoming of the transcription start site is CC at positions þ1 and þ2,to nucleotide and stabilizes a pentacovalent phosphate intermediate form Watson–Crick base pairs with two molecules of GTP upon during the reaction. Both metal ions are proposed to have octa- nucleotide loading, followed by a nucleotidyl transfer reaction 2þ hedral coordination at physiological Mg concentrations (4). to produce a 2-mer RNA—5′-pppGpG-3′—and a leaving PPi. During transcript elongation, RNAP carries out the loading of There are two molecules in the asymmetric unit and, in the cases a single nucleotide substrate at the N site followed by a nucleo- of the SCI, SCII, and MC, both molecules are quasi-identical. In tidyl transfer reaction with the RNA 3′ end at the P site; this cycle is repeated as elongation proceeds. X-ray crystal structures of the single-subunit T7 phage RNAP (2, 5) have depicted the process Author contributions: L.B.R.-D. and K.S.M. designed research; M.L.G., E.K.D., R.B., and K.S.M. performed research; M.L.G., E.K.D., R.B., L.B.R.-D., and K.S.M. analyzed data; of transcript elongation in detail and reveal a conformational and M.L.G., L.B.R.-D., and K.S.M. wrote the paper. change of the Fingers subdomain during substrate loading to the The authors declare no conflict of interest. active site as also observed in the A family of DNA polymerases (DNAPs) (6, 7). *This Direct Submission article had a prearranged editor. Initiation is the only step in the entire transcription process Data deposition: The atomic coordinates and structure factors have been deposited in the Protein Data Bank, www.pdb.org (PDB ID codes 3Q22 for substrate complex I, 3Q23 for where two nucleotide substrates are loaded at the active site substrate complex II, 3Q0A for the mismatch complex, and 3Q24 for the product complex). followed by a nucleotidyl transfer reaction. Compared with elon- 1Present address: Department of Biochemistry and Biophysics, School of Medicine and gation, the process of initiation has not been well characterized by Dentistry, University of Rochester, Rochester, NY 14642. X-ray crystallography. An X-ray crystal structure of T7 RNAP 2Present address: Department of Chemistry, University of Chicago, Chicago, IL 60637. initiation complex was reported (8), but it was captured by using 3To whom correspondence may be addressed. E-mail: [email protected] or lbrd@ a substrate analog 3′-deoxyGTP (Fig. S1B). This analog lacks the uchicago.edu. ′ essential O3 required for nucleotidyl transfer and catalytic metal This article contains supporting information online at www.pnas.org/lookup/suppl/ coordination resulting in the absence of the catalytic metal ion doi:10.1073/pnas.1016691108/-/DCSupplemental. www.pnas.org/cgi/doi/10.1073/pnas.1016691108 PNAS Early Edition ∣ 1of6 Downloaded by guest on September 29, 2021 A against the structure factors from the initiation complex crystals, F − F we observed clear unbiased o c electron densities around the active site, which corresponded to nucleotides and metals in the precatalytic complexes and a product 2-mer RNA plus PPi in the postcatalytic complex (Fig. 1 D–F). Compared to the binary complex, the backbone structures of the initiation B C complexes are almost identical (0.40 ∼ 0.65 Å rmsd) except for distinct deviations in the part of Fingers (residues 657–770, 1.5 ∼ 3.5 Å rmsd) including the O helix (residues 666–678) and DNA bases from −1 to þ2 (Figs. 2 and 3, and Movie S1). Structure of Substrate Complex I: Presence of Two Metals at the Active Site Is Essential for Catalysis. Precatalytic SCI (Figs. 1 A and D and 2B) was prepared by soaking 5 mM GTP and 10 mM MgCl2 into the BC crystals. SCI contained two molecules of GTP that base pair with DNA bases þ1 and þ2, and one Mg2þ ion as the nucleotide-binding metal. Mg2þ octahedrally coordinated with DEF ligands that include three atoms of the nonbridging triphosphate oxygens of GTPðþ2Þ, two carboxylates of the conserved Asp residues (D559 and D951), and the main-chain carboxyl group of G560 in the metal-binding motifs A and C that are common to the T7-like single-subunit RNAP family. The binding of the two GTP molecules and the Mg2þ to the BC triggers several conformational changes of DNA, the O helix of the Fingers and side-chain residues of motifs A and C in the Palm (Fig. 3A and Movie S1). Y678 at the O helix C terminus moves 4.3 Å to open the GTPðþ2Þ binding pocket and hydrogen bonds with the 2′-OH of GTPðþ2Þ. This movement is linked to a con- Fig. 1. The structure of the initiation complex. (A) A schematic representa- formational change of the O helix, which swings approximately tion of the sequential processes during initiation of transcription. The BC comprising RNAP and promoter DNA is depicted as “E,” and catalytic and nucleotide-binding metal ions are shown as MeA and MeB, respectively. (B) Sequences and secondary structures of the two DNA constructs used for crystallization. Regions highlighted by the gray boxes were disordered in the crystal structures. Nucleotide-binding sites (þ1 and þ2) for transcript initiation are colored in red. (C) Overall structure of the SCII. N4 mini-vRNAP is depicted as a molecular surface model.
Details
-
File Typepdf
-
Upload Time-
-
Content LanguagesEnglish
-
Upload UserAnonymous/Not logged-in
-
File Pages6 Page
-
File Size-