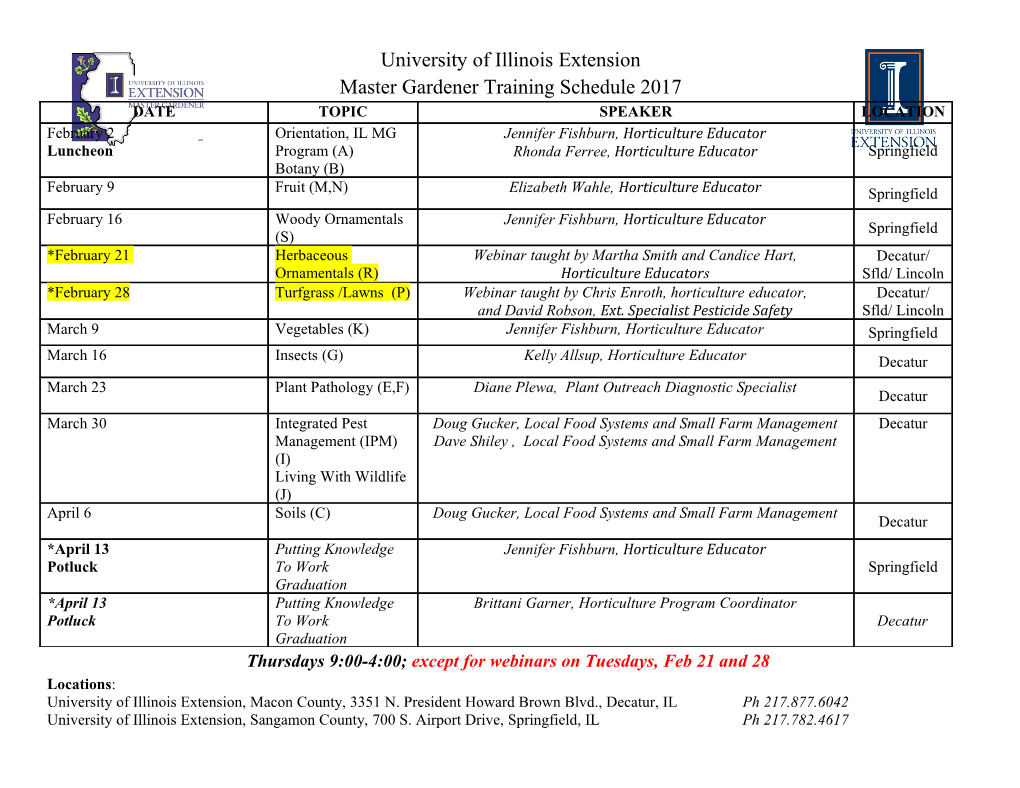
molecules Review Insights into the Structure and Energy of DNA Nanoassemblies § Andreas Jaekel 1, Pascal Lill 2, Stephen Whitelam 3 and Barbara Saccà 1,* 1 Zentrum für Medizinische Biotechnologi (ZMB), University of Duisburg-Essen, 45141 Essen, Germany; [email protected] 2 Structural Biochemistry, Max Planck Institute of Molecular Physiology, 44227 Dortmund, Germany; [email protected] 3 Molecular Foundry, Lawrence Berkeley National Laboratory, Berkeley, CA 94720, USA; [email protected] * Correspondence: [email protected] § Dedicated to Prof. Nadrian C. Seeman. Academic Editors: Magnus Willander and Peng Si Received: 27 October 2020; Accepted: 16 November 2020; Published: 24 November 2020 Abstract: Since the pioneering work of Ned Seeman in the early 1980s, the use of the DNA molecule as a construction material experienced a rapid growth and led to the establishment of a new field of science, nowadays called structural DNA nanotechnology. Here, the self-recognition properties of DNA are employed to build micrometer-large molecular objects with nanometer-sized features, thus bridging the nano- to the microscopic world in a programmable fashion. Distinct design strategies and experimental procedures have been developed over the years, enabling the realization of extremely sophisticated structures with a level of control that approaches that of natural macromolecular assemblies. Nevertheless, our understanding of the building process, i.e., what defines the route that goes from the initial mixture of DNA strands to the final intertwined superstructure, is, in some cases, still limited. In this review, we describe the main structural and energetic features of DNA nanoconstructs, from the simple Holliday junction to more complicated DNA architectures, and present the theoretical frameworks that have been formulated until now to explain their self-assembly. Deeper insights into the underlying principles of DNA self-assembly may certainly help us to overcome current experimental challenges and foster the development of original strategies inspired to dissipative and evolutive assembly processes occurring in nature. Keywords: DNA self-assembly; thermal annealing; DNA origami; nucleation; energy landscape 1. Introduction The discovery of the double-helical structure almost 70 years ago [1] is universally recognized as one of the pivotal points in the history of science. Since then, the DNA molecule has shown us its preeminent role in life, bridging the molecular level (i.e., the DNA and its protein products) with the system level of the cell and entire organism [2]. Most surprisingly, this task is accomplished through a simple four-letters code and one base-pair rule that provides DNA with a unique molecular feature—namely, self-recognition. In other words, the DNA carries in its structure the information for its own self-assembly, such that, given one strand, the complementary strand needed to form the double helix is uniquely defined. In the early 1980s, Ned Seeman recognized the tremendous implications of such a simple property for construction purposes and demonstrated how single-stranded DNA sequences can be programmed to associate into ordered molecular objects in a fully predictable fashion [3]. This led to the foundations of a new field of science, later known as structural DNA nanotechnology [4]. A notable breakthrough in the field occurred in 2006, when Paul Rothemund Molecules 2020, 25, 5466; doi:10.3390/molecules25235466 www.mdpi.com/journal/molecules Molecules 2020, 25, 5466 2 of 25 published a new method for folding DNA, called scaffolded DNA origami [5]. Despite relying on the same Watson-Crick base pairing rule, the DNA origami technique uses a long single-stranded DNA chain for the formation of the target shape and, by doing so, efficiently reduces the probability for spurious structures to form (we will see this process more in detail later). The consequence of this new building principle has been a dramatic improvement in our ability to engineer DNA nanomaterials, enabling us to gain access to structures with a level of sophistication previously unimaginable [6,7]. This, together with the availability of user-friendly design tools and the synthetic availability of oligonucleotides at relatively low costs, prompted the introduction of DNA nanotechnology into about 400 laboratories around the world and transformed a DNA-based building strategy into one of the methods of choice for controlling matter distribution with sub-nanometer precision [8–10]. Structural DNA nanotechnology relies on precise design rules. Thus, a deep understanding of the relationship between the topology of the DNA molecule and its mechano-elastic and thermal properties is necessary to construct objects that are more sophisticated than a canonical double helix. Not surprisingly, the pioneer of the field is a crystallographer, and his initial works on DNA branched motifs can be best appreciated and understood only if viewed in terms of DNA topology and related energetic stability. Fortunately, modern computer-aided programs have facilitated the accessibility of DNA design approaches to nonexperts; nevertheless, any effort devoted to the improvement of current strategies or the creation of new ones necessarily requires awareness of the underlying theoretical frameworks. Our purpose, in this review, is to give an overview of such theoretical background. We will therefore report in some detail the structural and energetic properties of DNA nanostructures and present the current hypotheses and models of the mechanisms that regulate their self-assembly. We will start by describing the DNA duplex (Section2) and will move to the basic building block of all DNA nanostructures—namely, the Holliday Junction (HJ), also called four-arm junction (Section3). We will survey both the structural and energetic properties of this fundamental motif and summarize the final view that has been acquired through numerous thermodynamic and kinetic studies, both in bulk and at the single-molecule level. In Section4, we will briefly review more complex DNA branched motifs, thus laying the ground for a better understanding of the self-assembly approach originally developed by Seeman, later called the multi-stranded (or tile-based) approach. We will then focus on the other main design school, i.e., the scaffold-based (or DNA origami) approach, and briefly discuss the structural and thermal properties of such objects (Section5). Finally, in Section6, we will present and critically compare both design strategies and discuss their analogy to the nucleation-and-growth mechanism of crystal formation. We will conclude by reporting recent examples of alternative approaches for guiding DNA self-assembly. In such methods, structural stability and dynamic reconfiguration properties are cleverly combined to enable the system to read information from the external environment and respond to a change, thus adapting to a new condition. These strategies, although still in their infancy, pave the way to a completely new paradigm of DNA self-assembly that—by mimicking nature—allows the system to undergo selection and, in this sense, to evolve. 2. The Double Helix A single-stranded DNA is a polynucleotide chain consisting of the nitrogen-containing nucleobases adenine (A), guanine (G), thymine (T) and cytosine (C), covalently linked to one another through a sugar-phosphate backbone. In its most common B-form, the DNA molecule is composed of two such strands oriented in an antiparallel fashion and wrapped together into a right-handed double helix, which is about 2-nm-wide and has a helical pitch of 3.4 nm every 10.5 base pairs (bp) (Figure1). Two main forces are responsible for formation of the double helix: (i) stacking among the aromatic rings of adjacent nucleobases along each strand and (ii) hydrogen bonding between the bases of opposite strands. Whereas the former mostly contributes to the stabilization of the structure, the latter is what drives the self-recognition process according to the Watson-Crick complementarity rule (A/T and G/C). Base pairing is therefore the feature that makes the DNA molecule an ideal semanto-morphic material, MoleculesMolecules2020 2020, 25, 25, 5466, x 33 ofof 2524 semanto-morphic material, i.e., a material that holds the information (semantic) for building its own i.e.,structure a material (morphology). that holds Most the information artificial DNA (semantic) nanostru forctures, building from the its ownimmobile structure Holliday (morphology). junction to MostGDa-sized artificial DNA DNA origami nanostructures, multimers from and the immobileDNA bric Hollidayks, are junctionbased on to GDa-sizedthe association DNA of origami DNA multimersoligonucleotides and DNA into bricks,double arehelical based segments on the an associationd loops intertwined of DNA oligonucleotides with one another into in doublevarious helicalways. The segments use of andalternative loops intertwineddouble helical with forms one (s anotheruch as Z-DNA) in various has ways. been limited The use to offew alternative examples, doublemost likely helical due forms to the (such requirement as Z-DNA) of non-physiologi has been limitedcal conditions to few examples, such as reduced most likely water due activity to the or requirementthe presence of non-physiologicalof metal-ion complexes conditions [11,12]. such as Other reduced DNA water secondary activity or thestructures, presence ofsuch metal-ion as G- complexesquadruplexes, [11,12 i-motifs,]. Other hairpin DNA dumbbells
Details
-
File Typepdf
-
Upload Time-
-
Content LanguagesEnglish
-
Upload UserAnonymous/Not logged-in
-
File Pages25 Page
-
File Size-