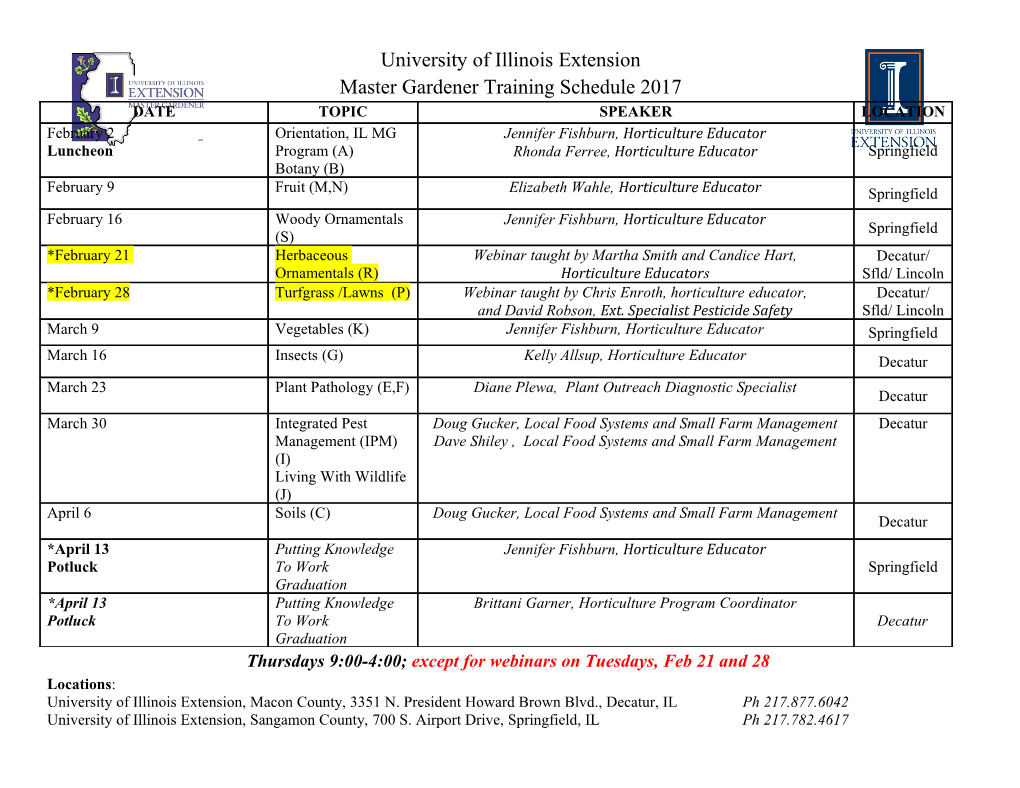
SEA LEVEL STUDIES/Isostasy 3043 Fleming, K., Johnston, P., Zwartz, D., Yokoyama, Y., Lambeck, Van Andel, T. H., and Veevers, J. J. (1967). Morphology and K., and Chappell, J. (1998). Refining the eustatic sea-level sediments of the Timor Sea. Bulletin of the Bureau of curve since the Last Glacial Maximum using far- and Mineral Resources, Geology and Geophysics, Australia. 83, intermediate-field sites. Earth and Planetary Science Letters 1–173. 163, 327–342. Veeh, H. H., and Veevers, J. J. (1970). Sea level at À175 m off the Goodwin, I. D. (2003). Unravelling climatic influences on Late Great Barrier Reef 13,600 to 17,000 years ago. Nature 226, Holocene sea-level variability. In Global change in the 536–537. Holocene (A. Mackay, R. Battarbee, J. Birks and F. Oldfield, Yokoyama, Y., De Deckker, P., Lambeck, K., Johnston, P., and Eds.), pp. 406–421. Arnold, London. Fifield, L. K. (2001). Sea-level at the Last Glacial Maximum: Hanebuth, T., Stattegger, K., and Grootes, P. M. (2000). Rapid evidence from northwestern Australia to constrain ice volumes flooding of the Sunda Shelf: A Late-Glacial sea-level record. for oxygen isotope stage 2. Palaeogeography, Palaeoclimatology, Science 288, 1033–1035. Palaeoecology 165, 281–297. Lambeck, K. (1995). Late Devensian and Holocene shorelines of the British Isles and North Sea from models of glacio-hyrdo- isostatic rebound. Journal of the Geological Society, London 152, 437–448. Lambeck, K., and Johnston, P. (1995). Land subsidence and Isostasy sea-level change: Contributions from the melting of the G Milne and I Shennan, Durham University, last great ice sheets and the isostatic adjustment of the Durham, UK Earth. In Land Subsidence (F.B.J.Barends,F.J.J. Brouwer and F. H. Schro¨ der , Eds.), pp. 3–18. Balkema, ª 2007 Elsevier B.V. All rights reserved. Rotterdam. Milne, G. A., and Mitrovica, J. X. (1998). Postglacial sea-level change on a rotating Earth. Geophysical Journal International 133, 1–19. Introduction Mitrovica, J. X., and Milne, G. A. (2002). On the origin of late Holocene sea-level highstands within equatorial ocean basins. Relative sea level (RSL) is defined as the height of the Quaternary Science Reviews 21, 2179–2190. ocean surface relative to the solid Earth (or ocean Murray-Wallace, C. V. (2002). Pleistocene coastal stratigraphy, floor) and can be defined at any location within the sea-level highstands and neotectonism of the southern Australian passive continental margin – a review. Journal of oceans. Changes in RSL are driven, therefore, by Quaternary Science 17, 469–489. processes that produce a height shift in either of Murray-Wallace, C. V., Ferland, M. A., and Roy, P. S. (2005). these two bounding surfaces. At any given location, Further amino acid racemisation evidence for glacial age, multi- RSL changes over a wide range of timescales with ple lowstand deposition on the New South Wales outer con- tinental shelf, southeastern Australia. Marine Geology 214, characteristic periods ranging from less than a second 235–250. to millions of years. This broad spectrum of time Nakada, M., and Lambeck, K. (1989). Late Pleistocene and variation reflects the variety of processes that drive Holocene sea-level change in the Australian region and mantle RSL changes: atmospheric circulation, ocean circula- rheology. Geophysical Journal 96, 497–517. tion, changes in the distribution of grounded ice, Oppenheimer, S. (1998). Eden in the East – The drowned conti- nent of southeast Asia, p. 560. Weidenfeld and Nicolson, lunar tides, deformation of the solid Earth associated London. with internal buoyancy forces (mantle convection), Peltier, W. R. (1998). Global glacial isostatic adjustment and and changes in the distribution of mass at the Earth’s coastal tectonics. In Coastal Tectonics (I. S. Stewart and surface (e.g., growth and melting of ice sheets, ero- C. Vita-Finzi, Eds.), Vol. 146, pp. 1–29. Geological Society, London. sion, and deposition of rocks). Peltier, W. R. (2002). On eustatic sea level history: Last Glacial During the Quaternary, the dominant component Maximum to Holocene. Quaternary Science Reviews 21, of the RSL change observed using proxy records was 377–396. driven by the accumulation and ablation of major ice Pirazzoli, P. A. (1991). World Atlas of Holocene Sea-Level sheets. There are a number of physical mechanisms Changes, Oceanography Series 58, p. 300. Elsevier, Amsterdam. associated with this glaciation-induced sea-level Pirazzoli, P. A. (1996). Sea-Level Changes: The Last 20 000 Years, change and these are described in the following sec- p. 211. John Wiley and Sons, Chichester. tion. Models that incorporate these mechanisms can Ryan, W., and Pitman, W. (1998). Noah’s Flood: the new scientific be employed to predict RSL changes, which can, in discoveries about the event that changed history, p. 319. Simon and Schuster, New York. turn, be compared to the observations. It is through Shennan, I. (1999). Global meltwater discharge and the deglacial this modeling procedure that researchers have been sea-level record from northwest Scotland. Journal of able to better understand the physical processes Quaternary Science 14, 715–719. involved in driving sea-level changes as well as Thom, B. G., and Chappell, J. (1975). Holocene sea levels relative place constraints on key model parameters. The to Australia. Search 6, 90–93. Thom, B. G., and Roy, P. S. (1985). Relative sea levels and coastal most common applications of this type will be pre- sedimentation in southeast Australia in the Holocene. Journal sented in the section ‘Applications of Sea-Level of Sedimentary Petrology 55, 257–264. Models.’ 3044 SEA LEVEL STUDIES/Isostasy Physical Principles of Glaciation-Induced deglaciate, this process is reversed and these so-called Sea-Level Change ‘peripheral bulges’ subside. The solid Earth deformation associated with the Vertical Deflection of the Solid Earth ice sheets, known as glacioisostasy, is by far the The late Quaternary ice sheets reached thicknesses of dominant signal in regions near major ice centers several kilometers in places. During a typical glacial (so-called ‘near-field’ regions). A significant amount cycle the net flux of mass from the oceans to the ice of deformation is also caused by the change in sea sheets and back again is on the order of 100 m of level associated with the change in ice distribution. mean global sea-level change. This redistribution of The solid Earth deformation associated with this surface water mass is large enough to cause a signifi- ocean loading, known as hydroisostasy, is most cant isostatic deformation of the solid Earth. This apparent in regions far from the major ice centers deformation is one of the important processes that (so-called ‘far-field’ regions) where the glacioisostatic contributes to glaciation-induced sea-level change signal is considerably reduced. For example, the (e.g., O’Connell (1971), Chappell (1974), Peltier small uplift around the periphery of Australia and and Andrews, (1976), and Clark et al. (1978)). Africa in Figure 1 is associated with the positive Figure 1 shows a model prediction of vertical ocean load applied around these continents during deformation at present associated with the most deglaciation. This uplift has been termed ‘continental recent deglaciation (ca. 20–7 cal kyr BP). The signal levering’ (e.g., Clark et al. (1978)). is dominated by crustal uplift in regions where large The surface mass redistribution and subsequent ice sheets once existed (e.g., North America, north- solid Earth deformation associated with Earth glacia- west Europe) or where the extent and thickness of ice tion perturbs the Earth’s inertia tensor and therefore was larger than at present (e.g., Antarctica). Even affects a change in Earth rotation: commonly though ice had disappeared in North America and described as a change in the spin rate (change in northwest Europe during the early- to mid-Holocene, length of day) and a change in the orientation of the there remains significant uplift in these regions due to spin axis relative to the solid Earth (True Polar the relatively high viscosity of mantle material, which Wander) (e.g., Sabadini et al. (1982) and Mitrovica limits the rate at which the solid Earth can attain et al. (2005)). The latter of these produces a signifi- isostatic equilibrium following the removal of ice. cant global-scale deformation of the Earth due to the Note that there are extensive regions of subsidence corresponding shift in the rotational potential. The peripheral to areas of uplift. This deformation is dominant geometry of this deformation is shown in linked to uplift of the solid Earth in regions periph- Figure 2. This rotation-induced pattern of deforma- eral to the growing ice sheets; when the ice sheets tion is relatively small (approximately 1–2 orders of t t+ –7.0 –2.0 –1.5 –1.0 –0.5 0.0 0.5 1.0 1.5 2.0 25.0 –1 Present-day radial deformation (mm yr ) Figure 2 Schematic diagram illustrating the geometry of the Figure 1 Predicted present-day vertical motion of the solid vertical solid Earth deformation driven by a clockwise wander of Earth based on a model of glacial isostatic adjustment. The ice the rotation pole between the times t and tþ. Shaded areas component of the model is adapted from the ICE-3G deglaciation indicate uplift and nonshaded areas indicate subsidence (asso- history (Tushingham and Peltier, 1991). Reproduced with permis- ciated with positive and negative perturbations to the rotational sion from Pugh D (2004) Changing Sea Levels: Effects of Tides, potential). Reproduced with permission from Mound JE and Weather and Climate, p. 280.Cambridge: Cambridge University Mitrovica JX (1998) True Polar Wander as a mechanism for Press. second-order sea-level variations. Science 279: 534–537. SEA LEVEL STUDIES/Isostasy 3045 magnitude less than the glacioisostatic signal).
Details
-
File Typepdf
-
Upload Time-
-
Content LanguagesEnglish
-
Upload UserAnonymous/Not logged-in
-
File Pages9 Page
-
File Size-