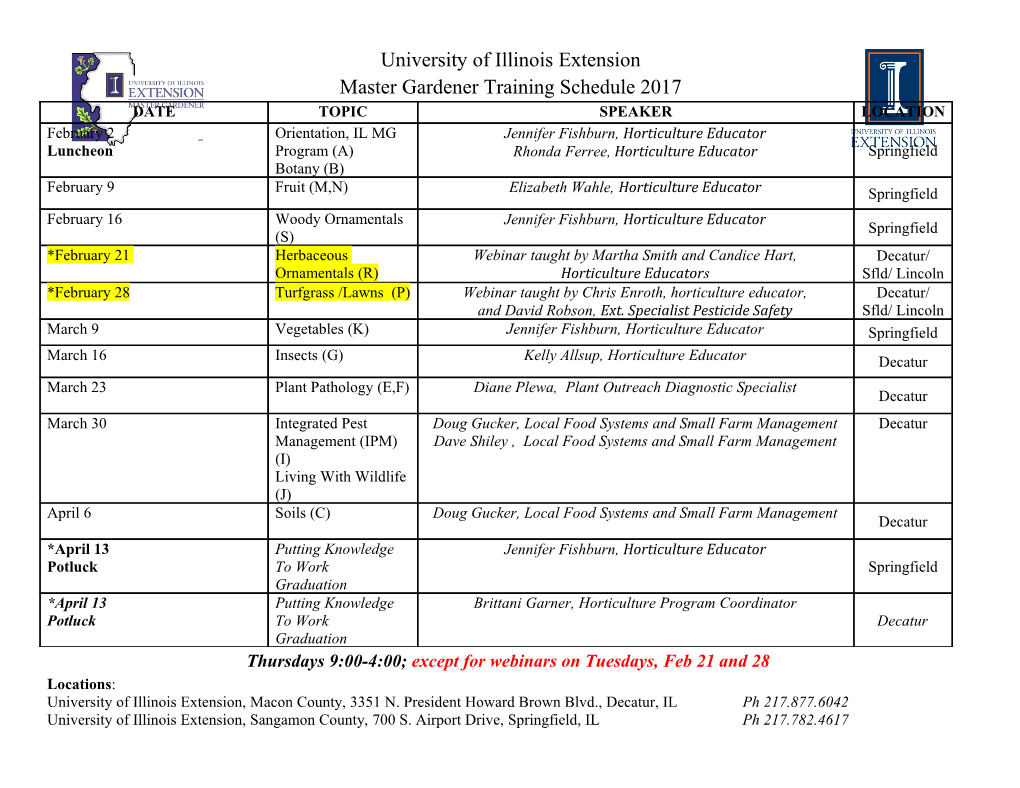
Water stable isotope spatio-temporal variability in Antarctica in 1960–2013: observations and simulations from the ECHAM5-wiso atmospheric general circulation model Sentia Goursaud, Valérie Masson-Delmotte, Vincent Favier, Anaïs Orsi, Martin Werner To cite this version: Sentia Goursaud, Valérie Masson-Delmotte, Vincent Favier, Anaïs Orsi, Martin Werner. Water stable isotope spatio-temporal variability in Antarctica in 1960–2013: observations and simulations from the ECHAM5-wiso atmospheric general circulation model. Climate of the Past, European Geosciences Union (EGU), 2018, 14 (6), pp.923-946. 10.5194/cp-14-923-2018. hal-02976236 HAL Id: hal-02976236 https://hal.archives-ouvertes.fr/hal-02976236 Submitted on 26 Oct 2020 HAL is a multi-disciplinary open access L’archive ouverte pluridisciplinaire HAL, est archive for the deposit and dissemination of sci- destinée au dépôt et à la diffusion de documents entific research documents, whether they are pub- scientifiques de niveau recherche, publiés ou non, lished or not. The documents may come from émanant des établissements d’enseignement et de teaching and research institutions in France or recherche français ou étrangers, des laboratoires abroad, or from public or private research centers. publics ou privés. Clim. Past, 14, 923–946, 2018 https://doi.org/10.5194/cp-14-923-2018 © Author(s) 2018. This work is distributed under the Creative Commons Attribution 4.0 License. Water stable isotope spatio-temporal variability in Antarctica in 1960–2013: observations and simulations from the ECHAM5-wiso atmospheric general circulation model Sentia Goursaud1,2, Valérie Masson-Delmotte1, Vincent Favier1, Anaïs Orsi2, and Martin Werner3 1Univ. Grenoble Alpes, CNRS, IGE, F-38000 Grenoble, France 2LSCE (Institut Pierre Simon Laplace, UMR CEA-CNRS-UVSQ 8212, Université Paris Saclay), Gif-sur-Yvette, France 3Alfred Wegener Institute, Helmholtz Centre for Polar and Marine Research, Bremerhaven, Germany Correspondence: Sentia Goursaud ([email protected]) Received: 25 September 2017 – Discussion started: 18 December 2017 Revised: 4 May 2018 – Accepted: 8 June 2018 – Published: 28 June 2018 Abstract. Polar ice core water isotope records are com- fer when using precipitation or ice core data. Further stud- monly used to infer past changes in Antarctic temperature, ies should explore the importance of deposition and post- motivating an improved understanding and quantification of deposition processes affecting ice core signals and not re- the temporal relationship between δ18O and temperature. solved in the model. This can be achieved using simulations performed by at- These results build trust in the use of ECHAM5-wiso mospheric general circulation models equipped with water outputs to investigate the spatial, seasonal, and inter-annual stable isotopes. Here, we evaluate the skills of the high- δ18O–temperature relationships. We thus make the first resolution water-isotope-enabled atmospheric general circu- Antarctica-wide synthesis of prior results. First, we show that lation model ECHAM5-wiso (the European Centre Ham- local spatial or seasonal slopes are not a correct surrogate for burg Model) nudged to European Centre for Medium-Range inter-annual temporal slopes, leading to the conclusion that Weather Forecasts (ECMWF) reanalysis using simulations the same isotope–temperature slope cannot be applied for the covering the period 1960–2013 over the Antarctic continent. climatic interpretation of Antarctic ice core for all timescales. We compare model outputs with field data, first with a fo- Finally, we explore the phasing between the seasonal cycles cus on regional climate variables and second on water stable of deuterium excess and δ18O as a source of information on isotopes, using our updated dataset of water stable isotope changes in moisture sources affecting the δ18O–temperature measurements from precipitation, snow, and firn–ice core relationship. The few available records and ECHAM5-wiso samples. ECHAM5-wiso simulates a large increase in tem- show different phase relationships in coastal, intermediate, perature from 1978 to 1979, possibly caused by a disconti- and central regions. nuity in the European Reanalyses (ERA) linked to the assim- This work evaluates the use of the ECHAM5-wiso model ilation of remote sensing data starting in 1979. as a tool for the investigation of water stable isotopes in Although some model–data mismatches are observed, the Antarctic precipitation and calls for extended studies to im- (precipitation minus evaporation) outputs are found to be prove our understanding of such proxies. realistic products for surface mass balance. A warm model bias over central East Antarctica and a cold model bias over 18 coastal regions explain first-order δ O model biases by too- 1 Introduction strong isotopic depletion on coastal areas and underestimated depletion inland. At the second order, despite these biases, The Antarctic climate has been monitored from sparse ECHAM5-wiso correctly captures the observed spatial pat- weather stations providing instrumental records starting at terns of deuterium excess. The results of model–data com- best in 1957 (Nicolas and Bromwich, 2014). Water stable parisons for the inter-annual δ18O standard deviation dif- isotopes in Antarctic ice cores are key to expanding the Published by Copernicus Publications on behalf of the European Geosciences Union. 924 S. Goursaud et al.: Water stable isotope spatio-temporal variability in Antarctica in 1960–2013 documentation of spatio-temporal changes in polar climate air mass trajectories. Thereupon, local temperature (i.e. at and the hydrologic cycle (Jouzel et al., 1997) for the recent a specific site) was reconstructed using δ18O measurements past (PAGES 2k Consortium, 2013; Stenni et al., 2017) as and based on the slope of the aforementioned spatial em- well as for glacial–interglacial variations (Jouzel et al., 2007; pirical relationship as a surrogate for relationships at annual Schoenemann et al., 2014). Water stable isotopes measured to multi-annual scales. However, recent data syntheses have along ice cores were initially used to infer Antarctic past tem- shown that other effects had to be taken into account (e.g. peratures using the spatial isotope–temperature slope (Lorius Masson-Delmotte et al., 2008). It was found that Antarc- et al., 1969). The focus on inter-annual variations is moti- tic snowfall isotopic composition is also linked to the ini- vated by the goal of quantifying temperature changes at the tial vapour isotopic composition (Stenni et al., 2016), atmo- Earth’s surface, including Antarctica, during the last millen- spheric transport pathways (Schlosser et al., 2008; Dittmann nia, to place current changes in the perspective of recent nat- et al., 2016), Antarctic sea ice extent (Bromwich and Weaver, ural climate variability (Jones et al., 2016), to understand the 1983; Noone and Simmonds, 2004; Holloway et al., 2016), drivers of this variability, and to test the ability of climate and local condensation temperature, which is itself related models to correctly represent it. This timescale is relevant for to surface temperature through complex boundary layer pro- the response of the Antarctic climate to e.g. volcanic forcing cesses (Krinner et al., 2007). Evaporation conditions, trans- and for the Antarctic climate fingerprint of large-scale modes port, and boundary layer processes may vary through time of variability such as ENSO and the Southern Annular Mode from seasonal (Fernandoy et al., 2018) to annual or multi- (Smith and Stearns, 1993; Turner, 2004; Stammerjohn et al., annual scale, thereby potentially distorting the quantitative 2008; Schroeter et al., 2017). The various climate signals po- relationship between snow isotopic composition and local tentially recorded in precipitation isotopic composition are, surface air temperature estimated empirically for present-day however, difficult to disentangle. conditions (Jouzel et al., 1997). First, the original signal from precipitation may be altered Model studies have been key to quantitatively exploring due to deposition and post-deposition processes (e.g. Sokra- the spatio-temporal aspects of the relationships between pre- tov and Golubev, 2009; Jones et al., 2017; Münch et al., 2017; cipitation isotopic composition and temperature (Jouzel et Laepple et al., 2018). Wind erosion and sublimation during al., 2000). Mixed-cloud isotopic models have been used to or after precipitation have long been known to affect ice core propose a coherent interpretation of δ18O and δD data in records (Eisen et al., 2008; Grazioli et al., 2017). Other pro- terms of changes in site and source temperatures (Uemura cesses such as melt and diffusion can also alter the preser- et al., 2012) or to simulate isotopic variations along individ- vation of isotopic signals in firn and ice and cause smooth- ual atmospheric trajectories (Dittmann et al., 2016). How- ing of the initial snowfall signals (Johnsen, 1977; Whillans ever, such theoretical distillation models rely on the clo- and Grootes, 1985; Johnsen et al., 2000; Jones et al., 2017). sure assumption at the ocean surface to calculate the initial So far, the mechanisms of such post-deposition processes evaporation isotopic composition and do not account for at- on the alteration of the initial precipitation signals are not mospheric dynamics and mixing of air masses (Jouzel and fully understood and quantified (Touzeau et al., 2017). Sec- Koster, 1996; Delmotte et al., 2000). Atmospheric general
Details
-
File Typepdf
-
Upload Time-
-
Content LanguagesEnglish
-
Upload UserAnonymous/Not logged-in
-
File Pages25 Page
-
File Size-