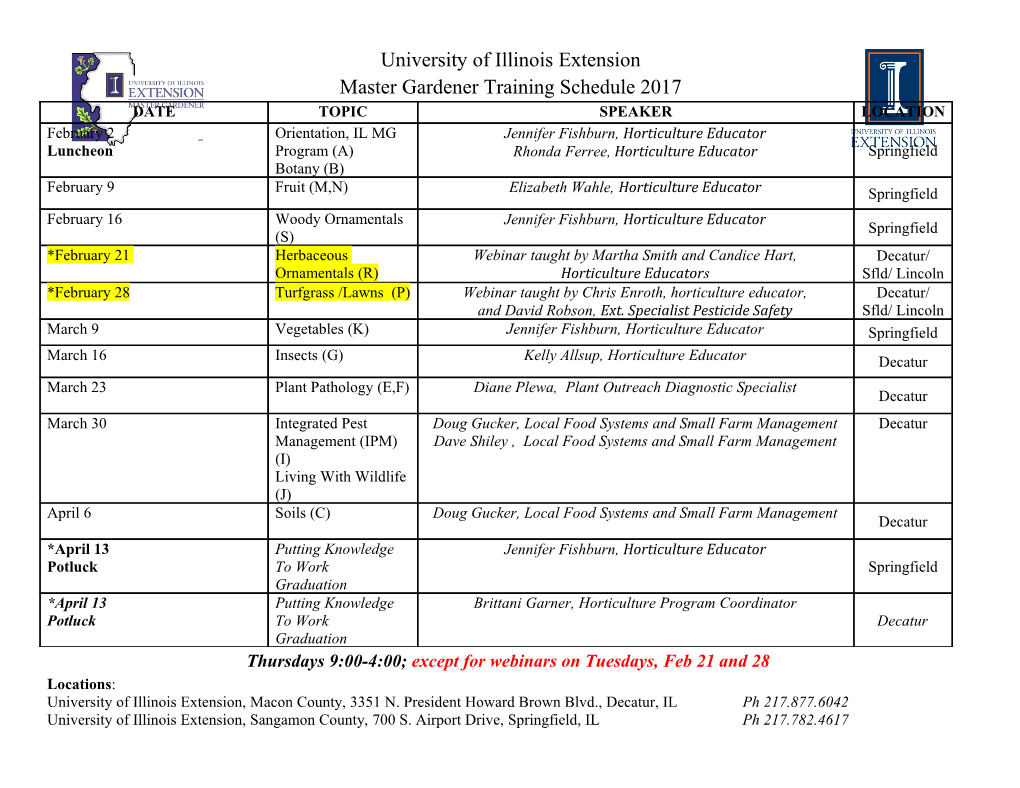
Université du Québec Institut National de la Recherche Scientifique Centre Énergie, Matériaux et Télécommunications Optical quantum state generation with integrated frequency comb sources Par Christian Reimer Thèse présentée pour l’obtention du grade de Philosophiæ Doctor, Ph.D. en sciences de l’énergie et des matériaux Jury d’évaluation Directeur de recherche Prof. Roberto Morandotti INRS-EMT Président du jury et Prof. Andreas Ruediger examinateur interne INRS-EMT Examinateur externe Prof. Marko Lončar Harvard University Examinateur externe Prof. Lilian Childress McGill University © Christian Reimer, 2017 ii Abstract The exploitation of quantum mechanics has the potential to solve important challenges and introduce novel technologies enabling, among others, powerful computation (which can solve certain problems exponentially faster than classical computers), perfectly secure communications, as well as sensing and imaging with precision and resolutions not achievable by classical means. In order to make use of the unique properties of quantum physics, experimental platforms that provide access to, and allow the control of quantum states, need to be developed. In particular, quantum systems composed of multiple parties are required, where each party is in a coherent superposition of states, and where multiples of such parties are in a coherent superposition with each other (e.g. being entangled). Additionally, in order to make use of such entangled quantum systems, it is required that each individual party can be coherently controlled and measured. The usability and available quantum resources of the entangled system is related to its size, which is proportional to the number of entangled parties, as well as the dimensionality of the superposition each party is in. For this reason, there is a imminent demand to achieve, investigate, and improve the generation and control of large quantum systems, comprised of as many entangled parties as possible, where each party is in an ideally high-dimensional superposition. Many quantum platforms are currently subject to extensive research, such as trapped ions, superconducting circuits, defect centers in solid-state crystals, mechanical oscillators, and optical quantum states (i.e. photons). While all platforms provide distinct advantages (as well as challenges), optical photon states are of particular interest, because they can interact with other quantum systems, and can be transmitted over long distances while preserving their quantum coherence without the need for extensive shielding. A large variety of sources for optical quantum states has been demonstrated, however most such implementations suffer from high complexity, ultimately limiting their scalability. Integrated photonics has recently become a leading platform for the compact, cost-efficient, and stable generation and processing of optical quantum states. However, to date, integrated quantum sources have only been able to generate photon pairs, which operated on single channels, and single polarization modes. Furthermore, in the few realizations of on-chip entanglement, they were limited to two entangled parties, where each party was in a two-dimensional superposition. These limitations of current integrated photon iii sources arise in large part from the concepts currently exploited to generate and control these states. In particular, path-entangled sources are the most commonly used, but increasing the quantum state complexity requires a significant, and very challenging, increase in device complexity. There is therefore an urgent need to develop new concepts that can achieve the on- chip generation of large and complex photon states without increasing source complexity, while still enabling coherent quantum state control and detection. In this work, we aim to address these limitations by making use of multiple frequency and temporal modes within a single spatial waveguide mode (therefore readily compatible with standard optical fibers) of an on-chip microring resonator. This approach is in contrast to more commonly used techniques relying on path entanglement, which exploits multiple spatial modes and generates entanglement by means of complex waveguide structures. Working within a single spatial mode, we achieve the first demonstration of pure heralded single photons and entangled photon pairs, which are emitted on multiple frequency modes from a single source. We then demonstrate the first generation of multi-photon entangled quantum states on a photonic chip. Finally, by making use of the spectral multi-mode nature of optical frequency combs, we achieve the generation of photon pairs in a coherent superposition of multiple frequency modes, representing the first generation of high-dimensional entangled states on a photonic chip. Our work represents a significant step forward in the generation of complex quantum states on a photonic chip and in a single spatial mode compatible with standard optical fibers. In addition, we demonstrated coherent quantum state control and manipulation for all generated states by means of standard telecommunications components, which could in the future be also integrated on single, monolithic chip. Our results indicate that the exploitation of integrated optical frequency comb sources for the generation of optical quantum states can provide a scalable and practical platform for quantum technologies. iv Acknowledgments I would like to firstly thank Prof. Roberto Morandotti for being my supervisor and for supporting me both academically and financially, as well as for providing me with many great opportunities. I am thankful for his suggestions, discussions, and for the freedom and support he gave me to pursue new ideas. I would like to thank the Natural Sciences and Engineering Research Council of Canada (NSERC) for their financial support in the form of a Vanier Canada Graduate Scholarship, as well as for their nomination and financial support to participate in the 2016 Lindau Nobel Laureate Meeting. I thank the INRS for financial support in the form of a tuition fee exemption, as well as for administrative support. Special thanks go to all members of the Ultrafast Optical Processing and Nonlinear Photonics Group at INRS. In particular, I would like to thank: Dr. Michael Kues for his guidance, supervision, discussions and help with the experimental work included herein; Dr. Lucia Caspani for her help, supervision, and guidance; Dr. Marcello Ferrera for many fruitful discussions, and for convincing me to pursue my Ph.D. studies at INRS; Piotr Roztocki for countless discussions and help with several experiments, including the implementation of active fiber interferometer stabilization; Stefania Sciara for help with theoretical work; Dr. Benjamin Wetzel for experimental help, including the implementation of computer controlled amplitude and phase filters; Dr. Yoann Jestin for support, discussions, and for translating the summary section of this thesis from English to French. Finally, I would like to give special thanks to Robin Helsten for maintaining all equipment in the laboratories, and for building countless custom mechanical and electronic components, without which the experiments would not have been possible. I would like to thank our external collaborators Prof. Sai T. Chu and Prof. Brent E. Little for providing us with state-of-the-art integrated photonic chips. Finally, I would like to thank my wife Nadia, as well as my parents Monika and Burkard for their constant support and love. Without your encouragement, patience and sacrifice I would not be where I am now. v Table of Contents I Abstract ………………………………………………………………………….. iii II Acknowledgements ……………………………………………………………… v III Table of Contents ………………………………………………………………... vi IV List of Figures …………………………………………………………………… viii V List of Tables ……………………………………………………………………. x VI List of Abbreviations ……………………………………………………………. xi VII Declaration of independent work, and overlap with publications ………………. xii 1. Introduction 1 1.1 General introduction …………………………………………………….. 1 1.2 Optical quantum states within a single spatial mode ……………………. 7 1.3 Objectives and thesis structure …………………………………………... 10 2. Methods and Techniques 12 2.1 Photon pair generation by means of nonlinear optical processes ………... 13 2.2 Nonlinear integrated optical microring resonators ………………………. 16 2.3 Optical excitation of microring resonators ………………………………. 18 2.3.1 Continuous wave excitation ……………………………………… 19 2.3.2 Pulsed excitation …………………………………………………. 20 2.4 Correlated photon detection measurements ……………………………… 22 2.4.1 Second-order photon coherence …………………………………. 24 2.5 Quantum interference ……………………………………………………. 27 2.5.1 Single photon or ‘classical’ interference ………………………… 27 2.5.2 Two-photon interference ………………………………………… 29 2.6 Quantum state tomography ……………………………………………… 32 2.7 Bell inequalities …………………………………………………………. 34 2.8 Entanglement in integrated microring resonators ……………………….. 37 2.8.1 Time-bin entanglement ………………………………………….. 37 2.8.2 Frequency-bin entanglement …………………………………….. 39 2.9 Novel technique for fiber interferometer stabilization …………………... 42 2.10 Novel technique for frequency-domain projection measurements ………. 46 vi 3. Results 49 3.1 Frequency comb of correlated photon pairs ……………………………... 50 3.1.1 Generation of multiplexed heralded single photons ……………... 50 3.1.2 Generation of cross-polarized photon pairs ……………………… 56 3.2 Frequency comb of entangled photon pairs ……………………………… 63 3.2.1 Generation of time-bin entangled photon pairs ………………….
Details
-
File Typepdf
-
Upload Time-
-
Content LanguagesEnglish
-
Upload UserAnonymous/Not logged-in
-
File Pages158 Page
-
File Size-