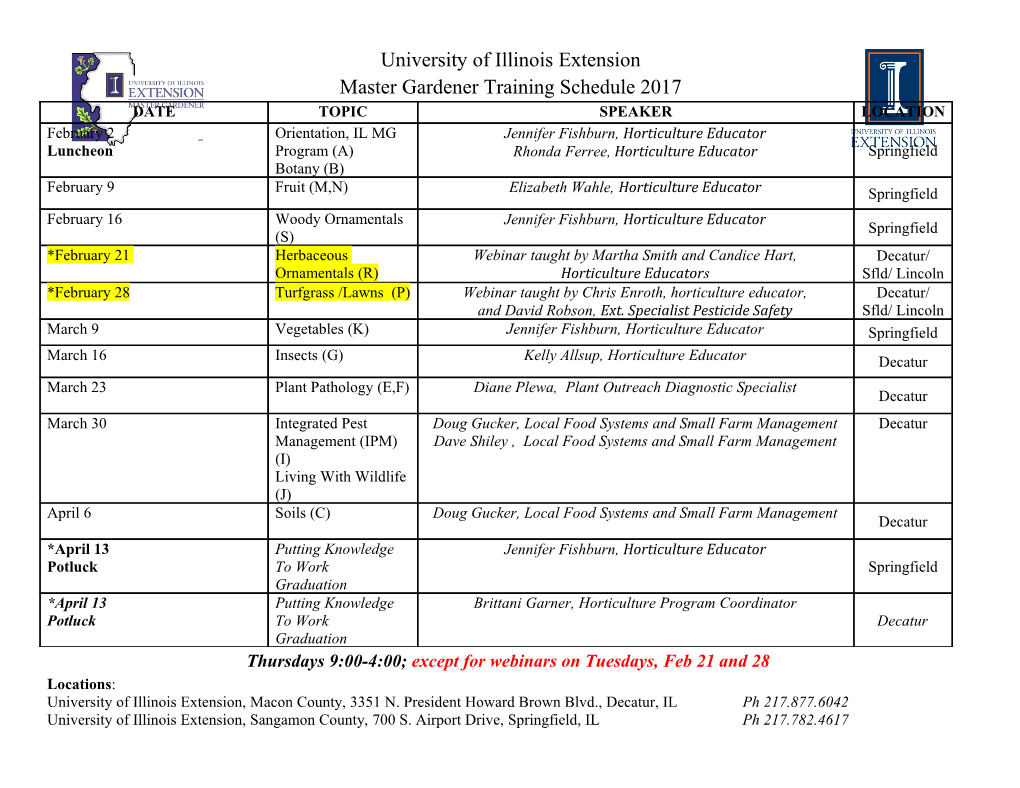
Not to be cited without prior reference to the authors ICES CM 2002/M:37 The Upper Ocean Circulation at Great Meteor Seamount. Part II: Retention Potential of the Seamount Induced Circulation ¡ Aike Beckmann and Christian Mohn Alfred Wegener Institute for Polar and Marine Research, Bremerhaven, Germany ¡ Max Planck Institute for Meteorology, Hamburg, Germany Abstract The circulation patterns at Meteor Seamount are investigated for implications for the marine ecosystem, using a numerical ocean circulation model. The importance of tidal rectification and internal tide generation has been documented in Part I of this study. Passive tracers confirm the idea that there is an area above the seamount which is largely isolated from the surroundings. Lagrangian particle trajectories are used to test and quantify the potential for retention. We find that passively advected organisms are more likely to remain in the near surface layers above Meteor Seamount than actively migrating organisms, who might escape from the area. Finally, the importance of strong wind events on the distribution of particles is illustrated. 1 Introduction 1.1 Aspects of Seamount Biology Marine biologists have been attracted to seamounts since the late fifties after the abundance of life above seamounts was discovered (Hubbs, 1959). Increased biomass and biodiversity are the characteristics of many seamounts, and the local conditions as well as their effects on larger scales have been investigated repeatedly. Comprehensive reviews were given by Boehlert and Genin (1987) and Rogers (1994). Our understanding of many of the above issues is still incomplete due to the large variety of physical and biological circumstances and the large effort that is necessary to investigate a seamount ecosystem as a whole. The effects of the oceanic circulation above seamounts and submarine banks on the distribu- tion of biogeochemical parameters have also been discussed frequently (e.g., Loder et al., 1988; Perry et al., 1993). Comeau et al. (1995) and Dower et al. (1992) discuss the relationship be- tween enhanced primary production and nutrient supply through locally upwelled water masses at Cobb Seamount in the northeast Pacific. Mullineaux and Mills (1997) found strong evidence that larvae of a wide range of benthic species are retained and aggregated by the tidally recti- fied three-dimensional flow system above Fieberling Guyot in the North Pacific. White et al. (1998) observed strong enrichment of nutrients over Porcupine Bank, a near-shore bank in the northeast Atlantic, located at the shelf edge west of Ireland. Summarizing our present knowledge of seamount biology, there are strong indications that the increased biomass and the level of endemism above many seamounts is profoundly in- fluenced by hydrodynamical processes and phenomena (upwelling, turbulent mixing, closed circulation cells). 1 Two aspects of the physical situation at the seamount seem particularly important for the biological system: the strength and persistence of closed circulation cells and the effect of the time-dependent flow field on individual particles. In this second part of our Meteor Seamount study we present the results from numerical process studies on the stability of the circulation. We simulate the advection and dispersion of passive particles to examine possible biological implications. Finally, we investigate the sensitivity of the flow system at the seamount to atmo- spheric forcing in terms of stability and stationarity. 1.2 Physical Elements of Seamount Regimes Strong currents and a generally enhanced variability have been observed frequently at seamounts. During a first systematic survey, the Atlantic Seamount Cruises 1967, it was noted that the hor- izontal homogeneity was strongly disturbed near the top of the seamount (Horn et al., 1971). A large number of studies have since confirmed the existence of strong long-period, sub-inertial and tidal currents at steep isolated topographic features at many locations in the world’s oceans (Huthnance, 1974; Hunkins, 1986; Genin et al., 1989; Codiga and Eriksen, 1997). Milestones in our theoretical understanding of flow around seamounts were works by Taylor (1917, 1923) on steady flow over topography, and the theory of freely propagating trapped waves at isolated topography by Chapman (1989) and Brink (1989, 1990). Numerical models have recently been used successfully to investigate the seamount regimes in the limit of steep slopes, tall topography and strong nonlinearity (Chapman and Haidvogel, 1992; Haidvogel et al., 1993; Beckmann and Haidvogel, 1997). Most investigations have fo- cussed on circular seamounts, and many phenomena can be exemplified in this prototype. 1.2.1 Taylor columns/caps Steady flow encountering a seamount leads to the formation of a closed anticyclonic (clockwise on the northern hemisphere) circulation cell atop the topographic obstacle. This phenomenon is called a Taylor column, or, in stratified fluid, a Taylor cap and the corresponding doming of isopycnals has been observed frequently above seamounts. A region of weak or vanishing currents is found in the center of such a circulation cell. This stagnation area would be an exceptional place for biological (in-)activity and sedimenta- tion. However, stagnant areas can be expected only in areas where mean flows are strong, and mesoscale variability and tidal currents are weak. This is the case only in a few places over the world ocean. 1.2.2 Trapped waves Periodically alternating flow (as from eddying or tidal motion) at sub-inertial ( ¢¤£¦¥ ) fre- quencies can generate trapped waves (so-called seamount trapped waves) at a seamount. The gravest mode of these seamount trapped waves is again a dipole of vertical velocities and den- sity anomalies rotating anticyclonically about the seamount. The corresponding horizontal flow features counterrotating cells. Seamount trapped waves and are bottom intensified with a typ- ical vertical scale determined by the strength of the stratification. A continuous excitation of 2 these waves at their characteristic frequency (e.g., by alternating tides) can lead to resonance effects, with substantial amplification (Haidvogel et al., 1993). In this case, nonlinearities may generate a residual current, which is also anticyclonic and has a similar structure as the that for steadily forced Taylor caps. Note however, that this residual flow is the result of and often masked by strong fluctuating currents. 1.2.3 Free waves At super-inertial frequencies (e.g., by tidal forcing), free waves are generated at the seamount flanks. These internal tides (with wavelengths of the order of the Rossby radius of deformation) can be excited on all flanks of a seamount, with some being reflected into the deeper ocean, some being transmitted into the shallower areas of the seamounts. The density perturbations caused by internal waves can be of substantial amplitude; in observations they may be found dominant. 2 The Meteor Seamount Regime 2.1 Biological Observations µ Chl a (HP LC) [ g/l] 0 0.175 0.05 0 0.15 0.2 .0 0.1 5 0.15 0.1 0.1 100 0.15 0.15 ] r 0.125 a 0.05 b 0 . d 0 [ 5 5 0 . e 200 0 0.1 r u s 0.0 s 5 e 0.075 r 0.0 P 5 300 w 0.05 0. e 05 0.05 i 0.05 V a t a D n a e 0.025 c 400 O 0 5 0 10 0 15 0 N Distance [km] S Figure 1: Chlorophyll-a concentration on a North South transect crossing the summit of Meteor Seamount (M. Kaufmann, pers. comm.). At Meteor Seamount, a number of biological observations have been carried out (Nellen, 1973, 1999), in conjunction with hydrographic and current measurements. During the latter cruise, enhanced concentrations of phytoplankton (K. von Brock¨ el, pers. comm.) but no signif- icant differences in zooplankton biomass (R. Diekmann, B. Martin, pers. comm.) between the seamount area and the surrounding ocean were found. Isopycnal doming was found on top of 3 the seamount (Nellen, 1999), as well as an isolated patch of chlorophyll in the upper thermocline (between 50 and 110 m depth) above the seamount’s summit plain (M. Kaufmann, pers. comm., see Fig. 1). In general, all biological observations show a high variability (patchiness) on hori- zontal scales smaller than the seamount. Thus the biological situation at Meteor Seamount seems to be largely comparable to other seamounts with shallow summits in the World’s Ocean (e.g., Cobb Seamount, Fieberling Seamount, Bowie Seamount; see also Genin et al. (1994)). However, it is unique with respect to its location at the critical latitude for diurnal tides and the high amplitudes of semidiurnal tides (see Mohn and Beckmann, 2002). Our goal here is to investigate the underlying mechanisms for biological tracer distribution and particle movement. The latter has been done for an idealized seamount by Goldner and Chapman (1997), assuming strong steady flow and relatively weak tides. At Meteor Seamount, the physical forcing features strong tides and a weak mean flow. 2.2 The Physical Situation 4000 3000 2000 1000 300 2000 3000 0 km 30 115 112 109 106 103 100 97 94 91 88 85 m Figure 2: Depth of the Upper Thermocline Layer (UTL) in the central model domain. Contour interval is 3 m. The wide variety of possible regimes (mean flow or tidally dominated, weakly or strongly stratified and rotating, abrupt or gently sloping, irregularly shaped) requires a detailed study for each seamount under investigation. Basically, the processes described in the Introduction section are generally valid, their relative strength, however, varies from seamount to seamount. The situation at Meteor Seamount was investigated by Mohn and Beckmann (2002), who found most of the above mechanisms and phenomena are at work here simultaneously. Basis for these experiments and analyses is the model configuration described in Part I of this study (Mohn and Beckmann, 2002). The primitive equation ocean general circulation model with terrain-following coordinates was configured as a periodic channel oriented northeast– 4 southwest.
Details
-
File Typepdf
-
Upload Time-
-
Content LanguagesEnglish
-
Upload UserAnonymous/Not logged-in
-
File Pages15 Page
-
File Size-