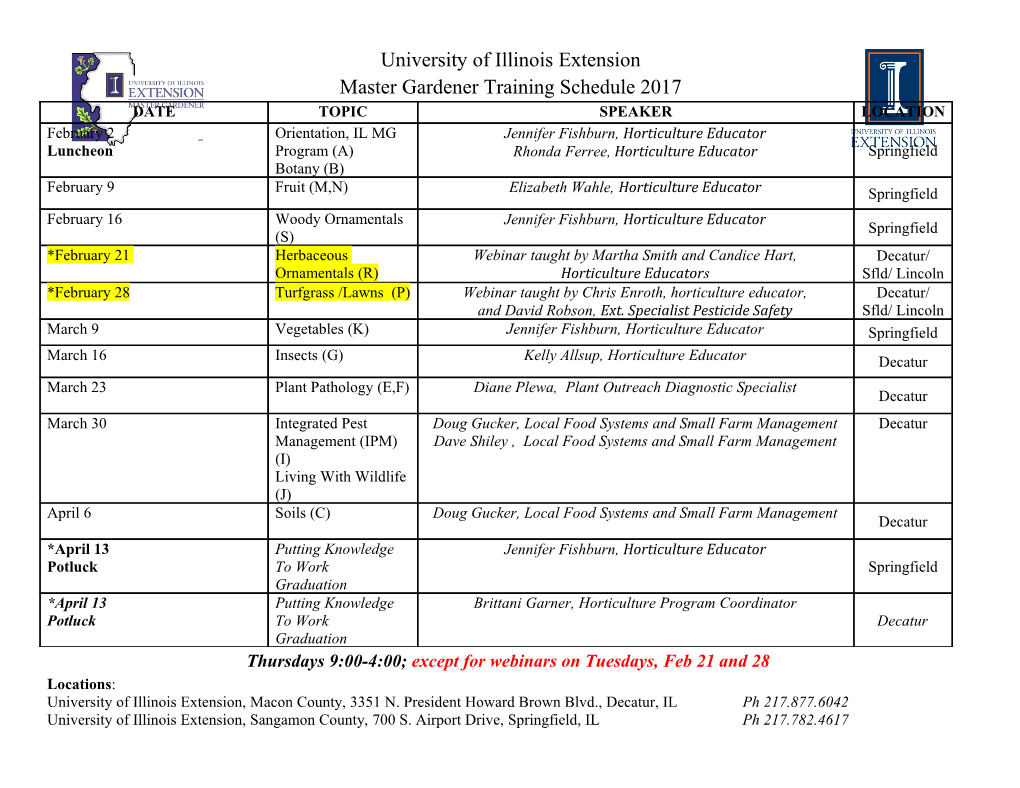
Top Quark Physics in the Large Hadron Collider era Michael Russell Particle Physics Theory Group, School of Physics & Astronomy, University of Glasgow September 2017 arXiv:1709.10508v2 [hep-ph] 31 Jan 2018 A thesis submitted in fulfilment of the requirements for the degree of Doctor of Philosophy Abstract We explore various aspects of top quark phenomenology at the Large Hadron Collider and proposed future machines. After summarising the role of the top quark in the Standard Model (and some of its well-known extensions), we discuss the formulation of the Standard Model as a low energy effective theory. We isolate the sector of this effective theory that pertains to the top quark and that can be probed with top observables at hadron colliders, and present a global fit of this sector to currently available data from the LHC and Tevatron. Various directions for future improvement are sketched, including analysing the potential of boosted observables and future colliders, and we highlight the importance of using complementary information from different colliders. Interpretational issues related to the validity of the effective field theory formulation are elucidated throughout. Finally, we present an application of artificial neural network algorithms to identifying highly- boosted top quark events at the LHC, and comment on further refinements of our analysis that can be made. 2 Acknowledgements First and foremost I must thank my supervisors, Chris White and Christoph Englert, for their endless support, inspiration and encouragement throughout my PhD. They always gave me enough freedom to mature as a researcher, whilst providing the occasional neces- sary nudge to keep me on the right track. I also have to thank David Miller for his friendly advice and irreverent sense of humour, and for helping me settle into the group in Glasgow, and Christine Davies, for financial support for several research trips, and for creating a wonderful place to do physics. This thesis would not have been written without the foundations laid down by Liam Moore, so I have to thank him for the countless hours he has put into the project, and for being a mate. Of the other students in Glasgow, I also have to mention Karl Nordstr¨omfor many useful conversations, both about the work presented here and often tangential (but always illuminating) topics, and his occasional computational wizardry. In Heidelberg, special mention must go to Torben Schell, for his patience in showing me some of the many ropes of jet substructure, and to Tilman Plehn for stimulating collaboration, and for helping me start the next chapter in my life as a physicist. I thank the students and postdocs in both of these places for making such a friendly working environment. Throughout my PhD I have been fortunate to work with several excellent experimen- talists; James Ferrando and Andy Buckley in Glasgow, and Gregor Kasieczka in Z¨urich. I thank them for all they have taught me about collider physics (and especially Andy for coding assistance in the rocky early days of TopFitter), and I hope to collaborate with them again in the future. Though I never had the opportunity to work with Sarah Boutle or Chris Pollard directly, their unwavering fondness for a pint helped keep me sane after many a long day. Finally, I have to acknowledge the huge intellectual (and general) debt I owe to my parents. Though they do not share my passion for physics, they have always supported me along the way, and politely endured far too many wearisome physics rants to mention. This thesis is dedicated to them. Thanks also to Daniel and Lucy for trying to keep me in the real world. 3 Declaration I declare that this thesis is the result of my own original work and has not been previously presented for a degree. In cases where the work of others is presented, appropriate citations are used. Chapters 1 and 2 serve as an introduction to the research topics presented in the rest of the thesis. Chapters 3 to 5 are a result of my own original work, in collaboration with the authors listed below. Work appearing in this thesis was presented in the following publications [1{5]: A. Buckley, C. Englert, J. Ferrando, D. J. Miller, L. Moore, M. Russell, and C. D. • White, \Global fit of top quark effective theory to data," Phys. Rev. D92 no. 9, (2015) 091501, arXiv:1506.08845 [hep-ph]. A. Buckley, C. Englert, J. Ferrando, D. J. Miller, L. Moore, M. Russell, and C. D. • White, \Constraining top quark effective theory in the LHC Run II era," JHEP 04 (2016) 015, arXiv:1512.03360 [hep-ph]. C. Englert, L. Moore, K. Nordstr¨om, and M. Russell, \Giving top quark effective • operators a boost," Phys. Lett. B763 (2016) 9{15, arXiv:1607.04304 [hep-ph]. A. Buckley, C. Englert, J. Ferrando, D. J. Miller, L. Moore, K. Nordstr¨om,M. Russell, • and C. D. White, \Results from TopFitter," in 9th International Workshop on the CKM Unitarity Triangle (CKM 2016) Mumbai, India, November 28-December 3, 2016. 2016. arXiv:1612.02294 [hep-ph]. G. Kasieczka, T. Plehn, M. Russell, and T. Schell, \Deep-learning Top Taggers or • The End of QCD?," JHEP 05 (2017) 006, arXiv:1701.08784 [hep-ph]. C. Englert and M. Russell, \Top quark electroweak couplings at future lepton collid- • ers," Eur. Phys. J. C77 no. 8, (2017) 535, arXiv:1704.01782 [hep-ph]. My specific contributions to these chapters are as follows. In chapter 3, I set up the EFT fitting framework, and used the Mathematica model developed by Liam Moore to simulate events, construct theory observables and derive confidence limits on the operators considered. In chapter 4, I wrote the boosted analysis and derived the various projected limits on the operators considered. All numerical results in those chapters were derived by myself. In chapter 5, my contributions involved generating events, writing the analysis code to construct the fat jets and subsequent images used, formulating the boosted decision tree used as a comparison, and helping to design the neural network architecture used. All figures in this thesis were generated by the author, with the following exceptions: Fig. 3.2 (A. Buckley), Figs. 4.13-4.14 (C. Englert), Fig. 5.5 and Figs. 5.7-5.9 (T. Schell). 4 5 Contents A historical introduction 10 1 The top quark in the Standard Model 19 1.1 Introduction . 19 1.2 The Standard Model of Particle Physics . 19 1.2.1 Before electroweak symmetry breaking . 20 1.2.2 The Higgs mechanism . 25 1.2.3 The Higgs mechanism in the Standard Model . 28 1.2.4 The parameters of the Standard Model . 31 1.3 Hadron collider physics . 34 1.3.1 Scattering theory . 34 1.3.2 Scale uncertainties . 35 1.3.3 The parton densities . 37 1.3.4 Standard Model parameters . 37 1.4 Top quark physics at hadron colliders . 39 1.4.1 Top pair production . 40 1.4.2 Charge asymmetries . 43 1.4.3 Single top production . 45 1.4.4 Higher-order processes . 49 1.4.5 Top quark decay . 50 1.5 Summary . 51 2 The top quark beyond the Standard Model 52 2.1 Introduction . 52 2.2 Motivations for physics beyond the Standard Model . 52 2.2.1 The hierarchy problem . 52 2.2.2 Vacuum stability . 56 2.2.3 Gauge coupling unification . 59 2.2.4 Strong CP violation . 60 2.3 The role of the top quark in specific BSM scenarios . 61 2.3.1 Low energy supersymmetry . 61 2.3.2 Little Higgs . 63 2.3.3 Warped extra dimensions . 65 2.4 Principles of effective field theory . 67 6 2.4.1 The Euler-Heisenberg Lagrangian . 67 2.4.2 Fermi theory of weak decay . 69 2.4.3 Renormalisation group treatment . 70 2.5 The Standard Model effective field theory . 72 2.5.1 D =5 .................................. 73 2.5.2 D =6 .................................. 74 2.6 The top quark sector of the Standard Model effective theory . 77 2.6.1 Top pair production . 78 2.6.2 Single top production . 82 2.7 Summary . 82 3 A global fit of top quark effective theory to data 84 3.1 Introduction . 84 3.2 Higher-dimensional operators . 85 3.3 Methodology . 86 3.3.1 Experimental inputs . 86 3.3.2 Treatment of uncertainties . 87 3.3.3 Fitting procedure . 88 3.4 Results . 92 3.4.1 Top pair production . 93 3.4.2 Single top production . 96 3.4.3 Associated production . 99 3.4.4 Decay observables . 101 3.4.5 Charge asymmetries . 102 3.4.6 Contribution of individual datasets . 104 3.5 Validity of the EFT approach . 106 3.5.1 Impact of quadratic terms . 106 3.5.2 Overflow bins . 108 3.5.3 Constraining UV models . 110 3.6 Summary . 112 4 Future prospects for top quark EFT 116 4.1 Introduction . 116 4.2 Improving the fit with boosted reconstruction . 117 4.2.1 Analysis details . 119 7 4.2.2 Results . 122 4.2.3 Interpreting the results . 125 4.2.4 Discussion . 127 4.3 Associated Z production projections . 128 4.3.1 Top electroweak couplings . 128 4.3.2 Total rates . 130 4.3.3 Impact of differential distributions . 131 4.4 Future collider prospects . 132 4.4.1 The tt¯ total cross-section . 134 4.4.2 Polarised beams . 137 4.4.3 CLIC constraints . 138 + 4.5 Beyond e e− tt¯: Precision Observables . 140 ! 4.5.1 Oblique corrections . 140 4.5.2 Non-oblique corrections . 141 4.6 Summary . 142 5 Top tagging with Deep Neural Networks 144 5.1 Introduction .
Details
-
File Typepdf
-
Upload Time-
-
Content LanguagesEnglish
-
Upload UserAnonymous/Not logged-in
-
File Pages220 Page
-
File Size-