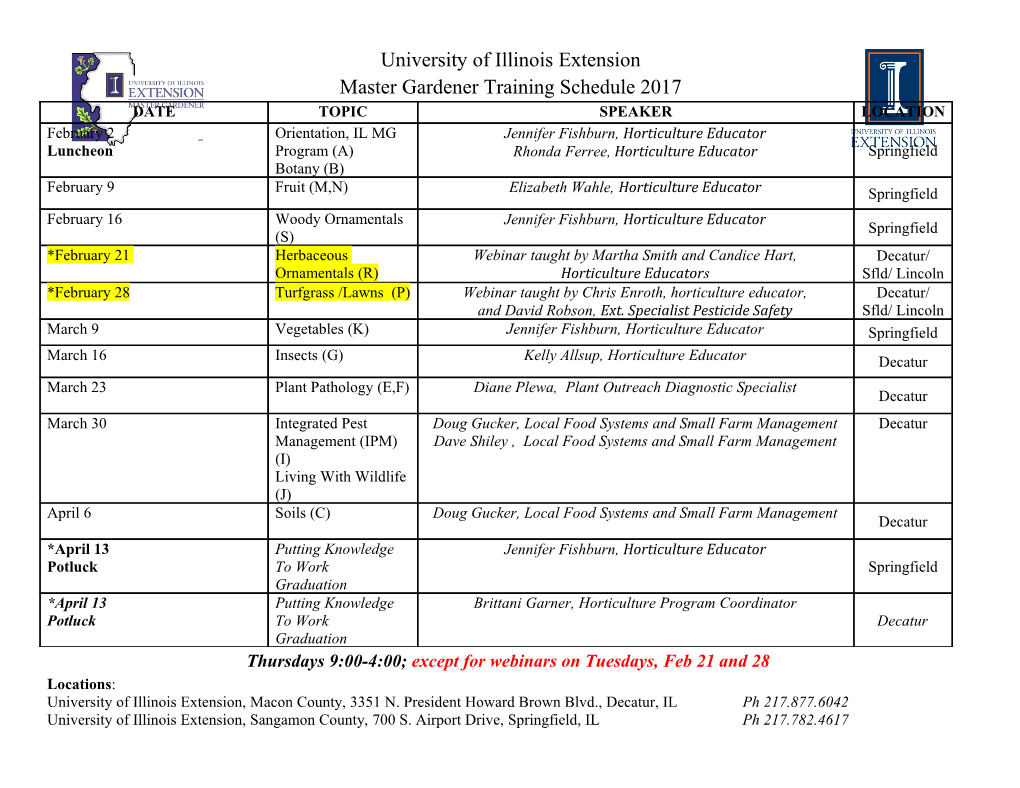
OPEN LETTER doi:10.1038/nature25014 Atmospheric trace gases support primary production in Antarctic desert surface soil Mukan Ji1*, Chris Greening2*, Inka Vanwonterghem3, Carlo R. Carere4, Sean K. Bay2, Jason A. Steen3, Kate Montgomery1, Thomas Lines2, John Beardall2, Josie van Dorst1, Ian Snape5, Matthew B. Stott4, Philip Hugenholtz3 & Belinda C. Ferrari1 Cultivation-independent surveys have shown that the desert Three surface soils were initially sampled from Robinson soils of Antarctica harbour surprisingly rich microbial Ridge (Wilkes Land)11 (Extended Data Fig. 1). Physicochemical communities1–3. Given that phototroph abundance varies across analysis showed that the soils were low in total organic carbon these Antarctic soils2,4, an enduring question is what supports (0.13 – 0.24%), nitrogen (0.012 – 0.023%), and moisture (4.0 – 5.6%) life in those communities with low photosynthetic capacity3,5. content (Supplementary Table 1). We used shotgun DNA sequencing to Here we provide evidence that atmospheric trace gases are the produce a 264-MB metagenome from these soils, comprising 208,233 primary energy sources of two Antarctic surface soil communities. predicted genes (Supplementary Table 2) and 451 predicted 16S/18S We reconstructed 23 draft genomes from metagenomic reads, rRNA operational taxonomic units (OTUs) (Supplementary Table 3). including genomes from the candidate bacterial phyla WPS-2 and The communities were dominated by Actinobacteria, Chloroflexi, AD3. The dominant community members encoded and expressed Proteobacteria, Acidobacteria, and two candidate phyla, AD3 and high-affinity hydrogenases, carbon monoxide dehydrogenases, WPS-2 (Fig. 1a). Identified phototrophs were limited to Cyanobacteria, and a RuBisCO lineage known to support chemosynthetic carbon with an average relative abundance of 0.28% (Fig. 1a). Consistent 6,7 5,11,14 fixation . Soil microcosms aerobically scavenged atmospheric H2 with other studies , the low photosynthetic capacity and organic and CO at rates sufficient to sustain their theoretical maintenance carbon content of Robinson Ridge soils contrasts with the inferred energy and mediated substantial levels of chemosynthetic but not bacterial diversity, suggesting that alternative energy sources support photosynthetic CO2 fixation. We propose that atmospheric H2, CO2 these communities. To gain a deeper understanding of ecosystem and CO provide dependable sources of energy and carbon to support function, we applied differential coverage binning15, which has been these communities, which suggests that atmospheric energy sources used to construct genomes of uncultured inhabitants of diverse envi- can provide an alternative basis for ecosystem function to solar or ronments15–18. We constructed 23 draft genomes from the Robinson geological energy sources8,9. Although more extensive sampling is Ridge metagenome, including two WPS-2 and three AD3 genomes required to verify whether this process is widespread in terrestrial (Fig. 1b; Supplementary Table 4). These candidate phyla affiliate with Antarctica and other oligotrophic habitats, our results provide new the Terrabacteria superphylum16 and are sister lineages to Chloroflexi understanding of the minimal nutritional requirements for life and and Armatimonadetes19 (Extended Data Fig. 2). open the possibility that atmospheric gases support life on other We subsequently analysed the metabolic potential of the metagenome planets. and derived genomes (Extended Data Fig. 3). All 23 sequenced organisms Terrestrial Antarctica is among the most extreme environments on harboured terminal oxidase genes to sustain aerobic respiration, con- Earth. Its inhabitants experience the cumulative stresses of freezing sistent with their aerated habitat, and genes for the oxidation of organic temperatures, limited carbon, nitrogen and water availability, strong UV carbon compounds were also abundant (Supplementary Tables 5, 6). 2,10,11 radiation, and frequent freeze–thaw cycles . Although it was once Genes supporting CO2 fixation through the Calvin–Benson–Bassham believed that these conditions restrict life, we now know that the con- (CBB) cycle were unexpectedly widespread, being found in the genomes tinent hosts a surprising diversity of macrofauna and microbiota1,2,12. of Actinobacteria, WPS-2, and AD3 (Fig. 1b, Supplementary Table 5). Surveys indicate that the phylum-level composition of microbial com- Phylogenetic analysis revealed that these genomes encoded the type IE munities in Antarctic soils is similar to those of temperate soils3, but ribulose-1,5-bisphosphate carboxylase (RuBisCO) (rbcL1E)6,7 (Fig. 2b, Antarctic communities are highly specialized at the species level and Extended Data Fig. 4a, b); this lineage is known to support hydrogeno- strongly structured by physicochemical factors1,3,10. In many Antarctic trophic growth of Actinobacteria7, but is absent from phototrophs. In soils, microorganisms are thought to live in dormant states2, with meta- addition, enzymes responsible for the aerobic respiration of mole- 13 20–22 23,24 bolic energy directed towards cell maintenance rather than growth . cular H2 and CO were encoded in multiple genomes (Fig. 1b, However, it is unclear how these communities obtain the energy and Supplementary Table 5). The high-affinity lineages identified—namely carbon needed for maintenance, given that these soils are often low the structural genes encoding group 1h [NiFe]-hydrogenases (hhyL in organic carbon and contain few classical primary producers2,5. and hhyS) (Fig. 2a, Extended Data Fig. 5a) and type I [MoCu]-carbon Cyanobacteria and algae are known primary producers in such monoxide dehydrogenases (coxL, coxS and coxM) (Extended Data 2,4 ecosystems , but multiple sites have now been described at which Fig. 6a)—support scavenging of H2 and CO at the trace concentra- phototrophs are present in low abundance or restricted to lithic tions found in the atmosphere21,25. We also identified determinants of niches5,11,14. These findings suggest that unidentified energy and methano trophy, ammonia oxidation, nitrogen cycling, and psychroto- carbon sources may also support ecosystem function. Here, we used lerance (Supplementary Tables 5, 7–9). shotgun metagenomics and biochemical measurements to clarify the Collectively, the metagenome data suggest that Antarctic surface basis of primary production in two such Antarctic sites. soil microbial communities have the capacity to scavenge H2, CO2 and 1School of Biotechnology and Biomolecular Sciences, Australian Centre for Astrobiology, UNSW Sydney, Randwick, New South Wales 2052, Australia. 2School of Biological Sciences, Centre for Geometric Biology, Monash University, Clayton, Victoria 3800, Australia. 3Australian Centre for Ecogenomics, School of Chemistry and Molecular Biosciences, The University of Queensland, St. Lucia, Queensland 4072, Australia. 4GNS Science, Wairakei Research Centre, 114 Karetoto Road, Wairakei, Taupo¯ 3384, New Zealand. 5Australian Antarctic Division, Department of Sustainability, Environment, Water, Population and Communities. 203 Channel Highway, Kingston, Tasmania 7050, Australia. * These authors contributed equally to this work. 400 | NATURE | VOL 552 | 21/28 DECEMBER 2017 © 2017 Macmillan Publishers Limited, part of Springer Nature. All rights reserved. LETTER RESEARCH a a Bin11, Actinobacteria (Solirubrobacterales) Soil 3 Bootstrap values Bin13, Dormibacteraeota (AD3) 1 Unbinned, sequence 119182 Soil 2 WP 041979300.1, Pyrinomonas methylaliphatogenes Unbinned, sequences 194515, 185456 Soil 1 Bin12, Dormibacteraeota (AD3) Unbinned, sequence 247823 0102030405060708090 100 0 Bin21, Verrucomicrobia (Chthoniobacterales) Relative abundance (%) Unbinned, sequence 79058 Bin8, Actinobacteria (Pseudonocardiales) Acidobacteria Cyanobacteria Eukaryotes Unbinned, sequence 91169 Dormibacteraeota (AD3) Planctomycetes Thaumarchaeota Proteobacteria Bacteroidetes Other bacteria Bin22, Eremiobacteraeota (WPS-2) Chloroexi Verrucomicrobia Unbinned, sequence 31136 Actinobacteria Eremiobacteraeota (WPS-2) WP 010988762.1, Streptomyces avermitilis WP 028930304.1, Pseudonocardia asaccharolytica Bin3,Bin4, Actinobacteria (Pseudonocardiales) b Unbinned, sequence 101038 Type IE RuBisCO 0.4 Bin11, Actinobacteria (Solirubrobacterales) Unbinned, sequences 138772, 79156, 266390 Type I [MoCu]-CO dehydrogenase WP 012813797.1, Robiginitalea biformata 0.3 WP 014512387.1, Sulfolobus islandicus Group 1h [NiFe]-hydrogenase 0.2 WP 011761956.1, Group 1g [NiFe]-hydrogenase Ammonia monooxygenase 0.1 0.1 Soluble methane monooxygenase b Unbinned, sequence 259512 Bin4, Actinobacteria (Solirubrobacterales) Type I NADH dehydrogenase 0 Bootstrap values 1 Unbinned, sequence 08379 Succinate dehydrogenase Bin3, Actinobacteria (Pseudonocardiales) WP 064080607.1, Rhodococcus opacus Cytochrome aa oxidase 3 Bin6, Actinobacteria (Pseudonocardiales) Bin12, Dormibacteraeota (AD3) Cytochrome cbb3 oxidase Unbinned, sequences 154260, 241641, 110540, 00264 Cytochrome bd oxidase 0 Unbinned, sequence 187302 WP 044200861.1, Oscillochloris trichoides F F -ATPase (ATP synthase) 1 o Unbinned, sequence 160018 WP 012852015.1, Thermomonospora curvata = 3) = 3) = 2) = 2) = 1) = 1) = 11) n n n n (n n WP 013678237.1, Pseudonocardia dioxanivorans (n ABC69587.1, Mycobacterium sp. DSM3803 Bin7, Actinobacteria (Pseudonocardiales) Chloroexi ( Unbinned, sequences 114617, 114329 Actinobacteria Bin22, Eremiobacteraeota (WPS-2) VerrucomicrobiaThaumarchaeota ( ( DormibacteraeotaEremiobacteraeota ( ( Bin13, Dormibacteraeota
Details
-
File Typepdf
-
Upload Time-
-
Content LanguagesEnglish
-
Upload UserAnonymous/Not logged-in
-
File Pages19 Page
-
File Size-