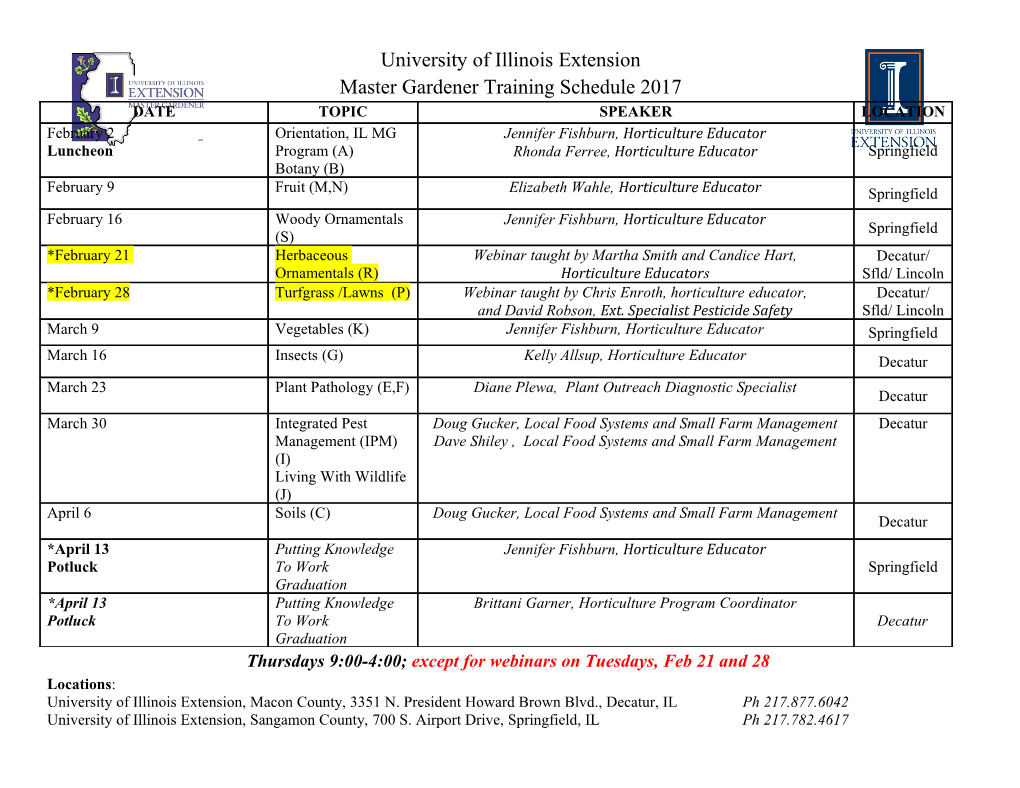
metals Article Low-Cycle Fatigue and Fracture Behavior of Aluminized Stainless Steel AISI 321 for Solar Thermal Power Generation Systems Wei Li 1,2, Lei Yang 1,2, Cong Li 1,2,*, Huitao Chen 1,2, Lu Zuo 1,2, Yide Li 1,2, Jian Chen 1,2, Jianjun He 1,2 and Sheng-de Zhang 3 1 Key Laboratory of Efficient & Clean Energy Utilization, School of Energy and Power Engineering, Changsha University of Science & Technology, Changsha 410114, China; [email protected] (W.L.); [email protected] (L.Y.); [email protected] (H.C.); [email protected] (L.Z.); [email protected] (Y.L.); [email protected] (J.C.); [email protected] (J.H.) 2 Department of Smart Grid, Hunan Province 2011 Collaborative Innovation Center of Clean Energy Institute, Changsha 410114, China 3 Japan Electric Power Central Research Institute, Tokyo 240-0196, Japan; [email protected] * Correspondence: [email protected]; Tel.: +86-137-8615-9450 Received: 27 July 2020; Accepted: 10 August 2020; Published: 12 August 2020 Abstract: The microstructure, low-cycle fatigue property, and fracture behavior of as-received and aluminized steel were investigated at room temperature, respectively. The results reveal that the aluminized layer is mainly composed of three layers: (I) the external Al2O3 layer, (II) the transition Fe-Al mesophase layer, and (III) the diffusion layer with AlFe and AlCrFe phase. The microhardness of as-received steel lower than that of aluminized steel until the distance from aluminized layer is greater than 150 µm. Compared to the original steel, the aluminized steel exhibits lower stress amplitude and fatigue life, which is correlated to the surface integrity. According to the Coffin-Manson relationship, the fatigue-ductility coefficients for as-received and aluminized steel is 4.347 and 3.528, respectively. Fractographic analysis reveals that the fatigue cracks tend to nucleate at the coating and propagate through the grain boundaries apace. Keywords: low-cycle fatigue; aluminized steel; surface integrity; fracture behavior 1. Introduction With the shortage of conventional fossil resource, the innovation of renewable energy sources has been facilitated by the increase of energy consumption unprecedentedly, which has attracted considerable interest [1]. Solar energy, as a major alternative energy sources, takes a large proportion in the total energy of the world [2]. There are two methods used for obtaining the solar energy. One is solar photovoltaic (PV) power generation and the other is concentrated solar thermal power (CSP) [3]. The latter is found to act as an important role in meeting national energy demands and reducing greenhouse gas emissions [4]. With the advantages of being relatively low cost and low emissions, CSP is more suitable to acquire stable dispatchable electrical output as compared to PV-based generation, especially in the application of utility-scale installations larger than 100 MW [5]. However, the interaction of thermal cyclic, mechanical stress and corrosion in heat transfer fluid medium would generate detrimental effect and accelerate the failure of heat exchange tube material [6–9], leading to an increase in the expenditure of maintenance. Therefore, it is very urgent to consider the mechanical strength of the materials as well as their capability against the environmental influence. AISI 321, a Ti-stabilized grade of austenitic stainless steel, is widely used as vital structural component material in CSP system, such as piping, heat exchangers, and receivers [10–12]. Whereas with Metals 2020, 10, 1089; doi:10.3390/met10081089 www.mdpi.com/journal/metals Metals 2020, 10, 1089 2 of 17 the application of diverse phase transformation heat storage materials, such as molten metal in which stainless steel is corroded seriously, it is inevitable for stainless steel to be refined and meet higher requirements in corrosion resistance. Therefore, it is extremely essential to develop an efficient and reliable coating for the structures of CSP system. Al2O3/Fe-Al coating is considered to achieve superior oxidation and corrosion resistance [13]. Different approaches such as chemical vapor deposition (CVD) [14], hot dip aluminizing [15], electrolytic deposition, and pack aluminizing [16] are adopted to prepare the aluminized layer. Among them, pack aluminizing is more prominent due to the cheap price and easy forming with different shapes and sizes [17]. The heat exchange tube material is mainly subjected to low cycle fatigue (LCF) loads under working condition. However, coating microstructure has an important effect on the fatigue performance of the steel. Mušálek R. [18] investigated the influence of Fe-Al coating prepared by plasma spraying on the fatigue behavior of low carbon steel, and found that the fatigue cracks initiated at coating-substrate interface and grew by striation mechanism. Additionally, several authors pointed out that the intrasplat cracking, decohesion, and the poor bonding ability of Al2O3 and Fe3Al would accelerate the fracture of material [19,20]. Furthermore, the surface integrity is of significance to the LCF life. Gun [21] investigated the effects of TiN coating on the fatigue life of AISI D2 tool steel and demonstrated that the fatigue limit was considerably related with the surface integrity. Moreover, many researchers reported that an increased microhardness can improve the LCF life [22–25]. However, there is still a shortage of systematical analyses about the interaction between microstructure and fatigue behavior in aluminized steel. Therefore, the microstructural evolution of aluminide coating and its effects on the LCF property are studied in this paper. The paper is organized as follows. Section2, after the aluminized steel is prepared, static tensile and LCF tests are conducted at room temperature. Subsequently, the microstructure, tensile property, surface integrity, hysteresis loops, cyclic stress response, and LCF life of aluminized steel are presented and compared with base metal in Section3. In the same section, the fatigue fractographic of these materials were observed using scanning electron microscopy (SEM). In Section4, the microstructure evolutions during pack aluminizing are discussed. Finally, the e ffective fatigue stress concentration and the degree of hardening for both steel are calculated. 2. Materials and Methods The nominal compositions of the stainless-steel (grade AISI 321) are shown in Table1. Test specimens were cut from the as-received slab and ground to 2000-grit SiC paper. They were rinsed with ethanol, and dried before aluminizing. Pieces of samples were completely embedded in the aluminizing agent, which is composed of 68 wt. % Fe-Al powder, 30 wt. % Al2O3 filler, and 2 wt. % NH4Cl activator, and then filled into a heat-proof stainless-steel container. The container was heated to 500 ◦C for 30 min primarily, and then elevated to 950 ◦C for 12 h at a heating rate of 10 ◦C/min, finally cooled to the room temperature in the air. Table 1. Chemical composition of 321 austenitic stainless steels. Element C Si Mn P S Cr Ni N Ti wt. % 0.035 0.38 1.08 0.028 0.003 17.02 9.06 0.045 0.22 All mechanical tests were performed on RDL05 electronic creep fatigue testing machine (Changchun Research Institute for Testing Machines, Changchun, China), equipped with 50 KN load cell. Static tensile tests and LCF tests were conducted at room temperature, using samples of 25 mm gauge length and 4 mm 8 mm sectional dimension as shown in Figure1. Tensile tests were × 3 1 implemented at a strain rate of 10− s− . LCF tests were carried out at a constant cyclic frequency of 0.2 Hz and the load ratio was R = 1. LCF tests were performed at total strain amplitudes of 0.3–0.8%. − All strain was measured by mounting extensometer. MetalsMetals 20202020,, 1010,, 1089x FOR PEER REVIEW 33 ofof 1717 Metals 2020, 10, x FOR PEER REVIEW 3 of 17 Figure 1. Schematic diagram of samples for tensile and fatigue tests, mm. Figure 1. SchematicSchematic diagram diagram of of samples samples for for tensile tensile and and fatigue fatigue tests, mm. The aluminized coatings were characterized by A TD3000 X-ray diffractometer (XRD, Dandong The aluminized coatings were characterized by A TD3000 X-ray diffractometer (XRD, Dandong TongdaThe aluminizedTechnology coatingsCo. Ltd, were Dandong, characterized China). by The A TD3000surface X-rayroughness diffractometer was measured (XRD, Dandongby JB-4C Tongda Technology Co. Ltd, Dandong, China). The surface roughness was measured by JB-4C Tongdaprecision Technology roughness testerCo. Ltd, and Dandong,3D surface China). profiles Thewere surface observed roughness through thewas VHX-1000 measured super-deep by JB-4C precision roughness tester and 3D surface profiles were observed through the VHX-1000 super-deep precision3D microscopic roughness system tester (Yalien and 3DInstitute surface of profilesMechanic wereal and observed Electrical through Technology, the VHX-1000 Beijing, super-deepChina). The 3D microscopic system (Yalien Institute of Mechanical and Electrical Technology, Beijing, China). 3Dmicro-hardness microscopic system was tested (Yalien with Institute a 410MVA of Mechanic Vickersal micro-hardness and Electrical Technology, tester (Laizhou Beijing, Huayin China). Institute The The micro-hardness was tested with a 410MVA Vickers micro-hardness tester (Laizhou Huayin Institute micro-hardnessfor Testing Instrument, was tested Laizhou, with a China) 410MVA using Vickers a load micro-hardness of 300 g and hold tester time (Laizhou of 15 s. HuayinFracture Institute surfaces for Testing Instrument, Laizhou, China) using a load of 300 g and hold time of 15 s. Fracture surfaces of forof theTesting fatigue Instrument, tested samples Laizhou, were China) examined using by a load Quanta of 300 2000 g and environment hold time SEMof 15 (FEI,s. Fracture Hillsboro, surfaces OR, the fatigue tested samples were examined by Quanta 2000 environment SEM (FEI, Hillsboro, OR, USA). ofUSA). the fatigue tested samples were examined by Quanta 2000 environment SEM (FEI, Hillsboro, OR, USA).3. Results 3. Results 3.3.1. Results Microstructure Characterization and Tensile Property 3.1. Microstructure Characterization and Tensile Property 3.1.
Details
-
File Typepdf
-
Upload Time-
-
Content LanguagesEnglish
-
Upload UserAnonymous/Not logged-in
-
File Pages17 Page
-
File Size-