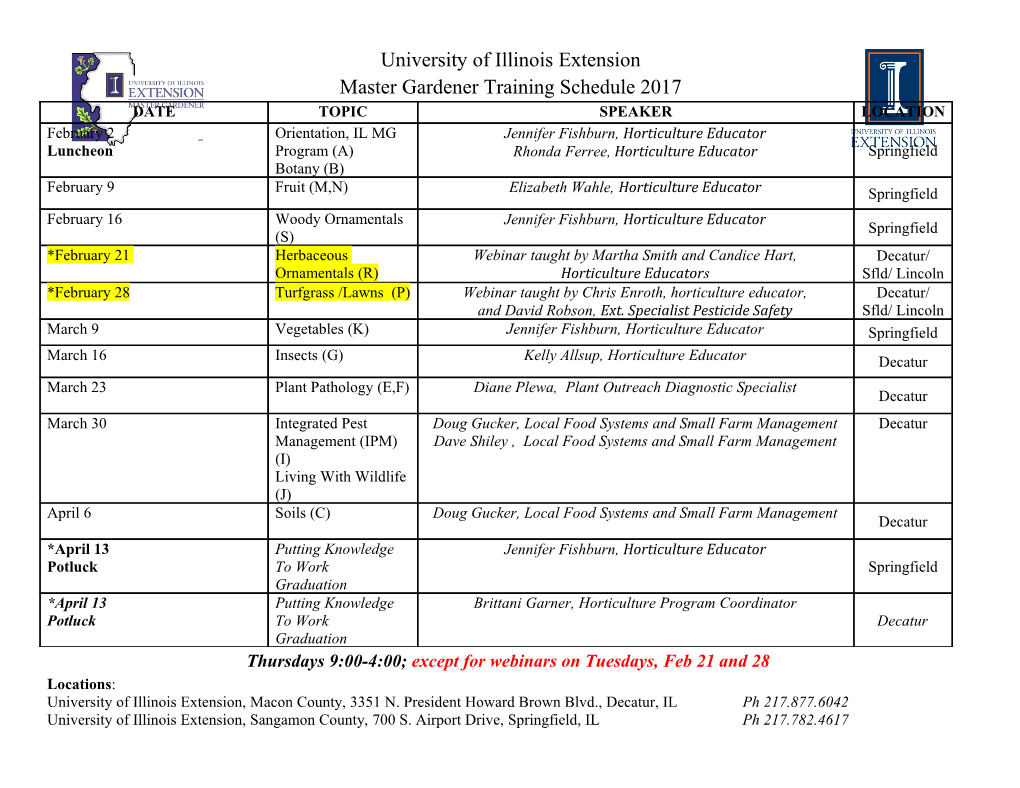
Metabolic Engineering 46 (2018) 13–19 Contents lists available at ScienceDirect Metabolic Engineering journal homepage: www.elsevier.com/locate/meteng Production of muconic acid in plants T ⁎ Aymerick Eudesa,b, , Roland Berthomieua,c, Zhangying Haoa,b, Nanxia Zhaoa,d, ⁎ Veronica Teixeira Benitesa,e, Edward E.K. Baidooa,e, Dominique Loquéa,b,f,g, a Joint BioEnergy Institute, EmeryStation East, 5885 Hollis St, 4th Floor, Emeryville, CA 94608, USA b Environmental Genomics and Systems Biology Division, Lawrence Berkeley National Laboratory, 1 Cyclotron Road, Berkeley, CA 94720, USA c Ecole Polytechnique, Université Paris-Saclay, Palaiseau 91120, France d Department of Bioengineering, Department of Chemical & Biomolecular Engineering, University of California, Berkeley, CA 94720, USA e Biological Systems and Engineering Division, Lawrence Berkeley National Laboratory, 1 Cyclotron Road, Berkeley, CA 94720, USA f Department of Plant and Microbial Biology, University of California, Berkeley, CA 94720, USA g Université Lyon 1, INSA de Lyon, CNRS, UMR5240, Microbiologie, Adaptation et Pathogénie, 10 rue Raphaël Dubois, F-69622, Villeurbanne, France ARTICLE INFO ABSTRACT Keywords: Muconic acid (MA) is a dicarboxylic acid used for the production of industrially relevant chemicals such as Muconic acid adipic acid, terephthalic acid, and caprolactam. Because the synthesis of these polymer precursors generates Salicylic acid toxic intermediates by utilizing petroleum-derived chemicals and corrosive catalysts, the development of al- Catechol ternative strategies for the bio-based production of MA has garnered significant interest. Plants produce organic Shikimate carbon skeletons by harvesting carbon dioxide and energy from the sun, and therefore represent advantageous Plastid hosts for engineered metabolic pathways towards the manufacturing of chemicals. In this work, we engineered Arabidopsis Arabidopsis to demonstrate that plants can serve as green factories for the bio-manufacturing of MA. In parti- cular, dual expression of plastid-targeted bacterial salicylate hydroxylase (NahG) and catechol 1,2-dioxygenase (CatA) resulted in the conversion of the endogenous salicylic acid (SA) pool into MA via catechol. Sequential increase of SA derived from the shikimate pathway was achieved by expressing plastid-targeted versions of bacterial salicylate synthase (Irp9) and feedback-resistant 3-deoxy-D-arabino-heptulosonate synthase (AroG). Introducing this SA over-producing strategy into engineered plants that co-express NahG and CatA resulted in a 50-fold increase in MA titers. Considering that MA was easily recovered from senesced plant biomass after harvest, we envision the phytoproduction of MA as a beneficial option to add value to bioenergy crops. 1. Introduction shikimate pathway for the biosynthesis of catechol precursors such as protocatechuate, anthranilate, salicylic acid (SA), and 2,3-dihydrox- Muconic acid (MA) is a platform chemical that serves as a precursor ybenzoic acid (Kruyer and Peralta-Yahya, 2017). Recently, MA bio- for the synthesis of products such as adipic acid, terephthalic acid, and synthetic pathways have been implemented in various microbial strains caprolactam which are widely used in the nylon and thermoplastic capable of growing in the presence of aromatics derived from lig- polymer industries. Current processes for the manufacturing of MA or nocellulosic biomass. These include engineered strains of Escherichia its derivatives mainly rely on non-renewable petroleum-based chemi- coli (Sonoki et al., 2014; Wu et al., 2017), Amycolatopsis sp. (Barton cals. Such processes are not sustainable and eco-friendly since they et al., 2017), Pseudomonas sp. (Vardon et al., 2015; Johnson et al., require a high energy input and yield large quantities of toxic by-pro- 2016, 2017; Sonoki et al., 2017), and Sphingobium sp. (Sonoki et al., ducts (Xie et al., 2014). 2017). As an alternative, the biological production of MA using engineered In addition to microbial synthesis, the metabolic engineering of microorganisms and inexpensive carbohydrate feedstocks has received photosynthetic organisms like plants also provides a sustainable ap- increasing attention over the past 20 years (Xie et al., 2014). Most of the proach for the production of valuable metabolites and materials established biological routes consist in the production catechol and its (Börnke and Broer, 2010; Farré et al., 2014). These chemicals, when subsequent conversion into MA by ring-cleaving catechol 1,2-dioxy- produced in engineered bioenergy and oilseed crops, represent value- genase (Vaillancourt et al., 2006). All these routes exploit the intrinsic added renewable co-products on top of the lignocellulose and seed oil Abbreviations: DAHP, 3-deoxy-D-arabino-heptulosonate; MA, muconic acid; SA, salicylic acid ⁎ Corresponding authors at: Environmental Genomics and Systems Biology Division, Lawrence Berkeley National Laboratory, 1 Cyclotron Road, Berkeley, CA 94720, USA. E-mail addresses: [email protected] (A. Eudes), [email protected] (D. Loqué). https://doi.org/10.1016/j.ymben.2018.02.002 Received 1 October 2017; Received in revised form 6 January 2018; Accepted 13 February 2018 Available online 21 February 2018 1096-7176/ © 2018 The Authors. Published by Elsevier Inc. on behalf of International Metabolic Engineering Society. This is an open access article under the CC BY license (http://creativecommons.org/licenses/BY/4.0/). A. Eudes et al. Metabolic Engineering 46 (2018) 13–19 1 A Glycolysis Fig. 1. Strategy used for the production of muconic PEP AroG* 234567Irp9 Irp9 NahG CatA acid in plants. (A) De novo biosynthetic pathway for DAHP DHQ DHS SHIK S3P EPSP CHA ISOCHA SA CAT MA muconic acid synthesis. Bacterial enzymes AroG*, Pentose phosphate Irp9, NahG, and CatA were expressed in Arabidopsis E4P Shikimate pathway pathway for the production of muconic acid from salicylic acid derived from the shikimate pathway. B pAtIRX5 pAtCCoAOMT pAtIRX8 pAtC4H Abbreviations are: CAT, catechol; CHA, chorismate; DAHP, 3-deoxy-D-arabino-heptulosonate; DHQ, de- pA6 (hyg ) schl1 irp9 schl2 aroG* pTKan (kan ) schl3 catA schl3 nahG hydroquinate; DHS, dehydroshikimate; E4P, ery- throse 4-phosphate; EPSP, 5-enolpyruvoylshikimate 3-phosphate; F6P, fructose 6-phosphate; G3P, glyceraldehyde 3-phosphate; ISOCHA, isochorismate; MA, muconic acid; PEP, phosphoenolpyruvate; PYR, pyruvate; S3P, shikimate 3- phosphate; SA, salicylic acid; SHIK, shikimate. The enzymes are as follows: 1, enolase; AroG*, feedback-resistant DAHP synthase (L175Q); 2, DHQ synthase; 3, DHQ dehydratase; 4, shikimate dehydrogenase; 5, shikimate kinase; 6, EPSP synthase; 7, chorismate synthase; Irp9, bifunctional ISOCHA synthase / ISOCHA pyruvate lyase; NahG, salicylate hydroxylase; CatA, catechol 1,2-dioxygenase. (B) Binary vectors used in this study. Boxes labelled “schl” denote plastid transit peptides. pA6 and pTKan denote the two binary vector backbones conferring hygromycin (hygR) and kanamycin (kanR) resistance in plants. Abbreviations are: schl1, plastid transit peptide from Arabidopsis ferredoxin2 (At1g60950); schl2, plastid transit peptide from pea (Pisum sativum) ribulose-1,5-bisphosphate carboxylase small subunit (GenBank: AAG45569.1); schl3, plastid transit peptide sunflower (Helianthus annuus) ribulose-1,5- bisphosphate carboxylase small subunit (UniProtKB/Swiss-Prot: P08705.1). pAtIRX5, pAtCCoAOMT, pAtIRX8, and pAtC4H designate the promoters of Arabidopsis cellulose synthase 4 (At5G44030), caffeoyl coenzyme A O-methyltransferase 1 (At4G34050), galacturonosyltransferase 12 (At5G54690), and cinnamate 4-hydroxylase (At2G30490) genes, respectively. used to generate energy (Snell et al., 2015). Because plants are auto- construct (Fig. 1B), the attB1-schl1-irp9-attB2 sequence was amplified trophs able to capture solar energy, they represent an attractive chassis by PCR to replace the Gateway attB2 recombination site (3’-end) by an for implementing de novo metabolic pathways for cost-effective pro- attB4 recombination site, and cloned into the Gateway pDONR221- duction of important chemicals (Yuan and Grotewold, 2015). P1P4 entry vector by BP recombination (Life technologies, Foster City, In plants, the shikimate pathway is confined to plastids and provides CA) to produce a pDONR221-L1-schl1-irp9-L4 construct. A chimeric the precursors for the synthesis of aromatic amino acids and derived DNA construct was synthesized (GenScript, Piscatway, NJ): it was metabolites, vitamins K1 and B9, and SA (Maeda and Dudareva, 2012). flanked by the gateway sequences attB4r (5’-end) and attB3r (3’-end), We therefore used Arabidopsis as a model system to investigate a novel and contained the tG7 terminator and a 2.2-Kb sequence corresponding bio-based approach for the phytoproduction of MA. In particular, the to the Arabidopsis CCoAOMT1 (At4g34050) promoter (pCCoAOMT). SA pool derived from chorismate via the shikimate pathway was con- This attB4r-tG7-pCCoAOMT-attB3r construct (Supplementary Data S1) verted to catechol and MA by dual expression and plastid-targeting of was then subcloned into the Gateway pDONR221-P4rP3r entry vector bacterial salicylate hydroxylase (NahG) and catechol 1,2-dioxygenase by BP recombination (Life technologies, Foster City, CA) to produce (CatA) (Fig. 1A). Additional supply of SA to the MA pathway was pDONR221-L4R-tG7-pCCoAOMT-L3R. A gene sequence encoding feed- achieved via the expression of bacterial salicylate synthase Irp9 and back-insensitive AroG (L175Q) from E. coli (NCBI Reference Sequence: feedback-resistant
Details
-
File Typepdf
-
Upload Time-
-
Content LanguagesEnglish
-
Upload UserAnonymous/Not logged-in
-
File Pages7 Page
-
File Size-